TiO2ナノ流体に向けて—パート2:アプリケーションと課題
要約
ナノ流体に関する研究は、熱または大量輸送における魅力的な特性、流動性、およびエネルギーシステムアプリケーション(ソーラーコレクター、冷凍、ヒートパイプ、エネルギー貯蔵など)の分散安定性により、爆発的に増加しています。レビューのこの第2部では、TiO 2 の適用に関する最近の研究を要約しています。 ナノ流体であり、TiO 2 をさらに探索するための課題と機会を特定します。 ナノ流体。 2つの徹底的なレビューは、研究者がTiO 2 の研究状況に関する知識を更新するための有用なリファレンスガイドになることが期待されます。 ナノ流体、および重要なコメント、課題、および推奨事項は、将来の研究の方向性に役立つ可能性があります。
<セクションデータ-タイトル=" レビュー ">レビュー
背景
最初の部分では、準備、安定性、および特性に関する研究をレビューしました。ナノ流体の調製の方向性と特性について多くの研究が行われていることがわかります[1,2,3,4,5,6,7]。一方、特にエネルギーシステムでのナノ流体の応用のために行われた多くの試みもあります[8、9、10、11]。熱および物質移動プロセスの強化により、TiO 2 ナノ流体は、ソーラーコレクター[12]、冷凍[13,14,16]、エネルギー貯蔵[17、18]、ヒートパイプ[19,20,21]、およびその他のエネルギー用途[22,23]の分野に暫定的に適用されています。 、24、25、26、27、28、29、30、31、32、33、34]、たとえば車のラジエーター[31]、PV / Tハイブリッドシステム[32、33]、および熱電併給(CHP )システム[34]。以前の研究では、TiO 2 の熱伝達特性 熱伝導、強制対流沸騰熱伝達、および自然対流熱伝達におけるナノ流体が要約されています[35]。ただし、TiO 2 のアプリケーションに関する包括的な要約にはほど遠いです。 ナノ流体; TiO 2 には多くの実用的なアプリケーションもあります ナノ流体。ここでは、パート2で、TiO 2 の熱伝導率とエネルギー関連のアプリケーションに関する詳細なレビューを提供します。 ナノ流体。以前のレポート[35]と組み合わせた2つのレビューが、TiO 2 の研究の進捗状況に関する包括的な理解を提供することを願っています。 ナノ流体。ナノ流体技術の開発により、ナノ流体はこれらのエネルギーシステムの新しい効率的な作動油として実際に適用されることが期待されています。
熱伝導率の向上への応用
ナノ流体の卓越した性能は、一般にナノ粒子を添加した流体の物理的特性に起因するため、ナノ流体の熱伝導率に関する実験的または理論的調査は、ナノ流体の分野で重要なトピックになるはずです。ほとんどの総説では、物性部分に熱伝導率が導入されていますが、熱伝導率を高めることも、ナノ流体の重要なアプリケーションの側面です。アプリケーション部分に熱伝導率を入れるもう1つの理由は、2つのレビューの内容のバランスを取るためです。
多くの実験的および理論的研究結果は、ナノ粒子の添加が流体の熱伝導率を明らかに改善できることを示しています。ナノ流体の熱伝導率に影響を与える要因は、次のグループとして誘発できます。(1)粒子の種類、含有量[36、37]、サイズ[38]、形状[39]、構造[40]などの内部要因。およびベース流体のタイプ[41]および可能性のある界面活性剤またはpH調整剤[42、43](ある場合)。 (2)温度[40]、超音速振動時間[44]、保管時間[45]、または実行時間[46]を含む外部要因。 (3)ナノ粒子の表面電荷状態[47]、粒子のクラスター[48]、界面ナノ層[49]、ブラウン運動[50]、凝集[51]、界面熱抵抗、質量などの微小宇宙要因差散乱[52]。以前の調査では、TiO 2 の熱伝導率を示す表が提供されています。 ナノ流体[35]。ただし、影響度に対するさまざまな影響要因を理解することは直感的でなく、不便です。したがって、このパート2では、TiO 2 の熱伝導率への影響 ナノ流体は、より知覚的な理解を提供するために図に示されています。
パーティクルの読み込み効果
TiO 2 の熱伝導率の増分の要約 –入手可能な文献にあるナノ粒子の体積分率を含む水ナノ流体を図1に示します。すべての実験結果から、TiO 2 ナノ粒子は、ベース流体の熱伝導率を高めることができます。ただし、さまざまな研究の増分は大きく異なります。たとえば、ナノ流体の熱伝導率の向上の1つは、TiO 2 の体積負荷の約2〜4倍です。 増田らを含むナノ粒子。 [53]、Turgut etal。 [54]、張等。 [55]、Wang etal。 [56]、Pak and Cho [57]、Yang etal。 [58]、およびMushed etal。の[59]の結果。もう1つの機能強化は、TiO 2 の6〜20倍の体積負荷に達する可能性があります。 Yooらを含むナノ粒子。 [60]、Wen and Ding [61]、Mushed etal。 [62]、彼等。 [63]、Chen etal。 [64]、およびSaleh etal。の[65]の結果。
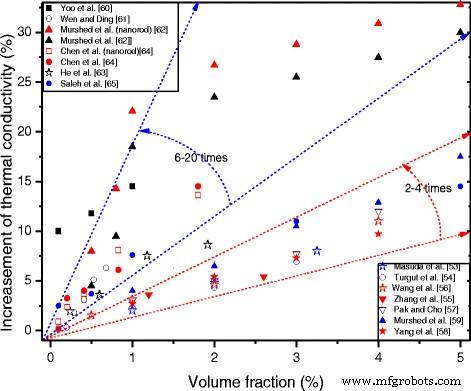
TiO 2 の熱伝導率の体積分率依存性 –入手可能な文献の水ナノ流体
結果の違いは、体積分率に加えて、TiO 2 の熱伝導率の結果である可能性があります。 ナノ流体は、粒子のパラメータと、粒子のサイズと形状、界面活性剤、pH値、温度などの環境環境によっても決定されます。これらは、作業ごとにまったく異なります。さらに、一部の研究者は、ナノ粒子がTiO 2 の熱伝導率にほとんど影響を与えないことを観察しました。 ナノ流体。 Utomo etal。 [66]は、水性アルミナおよびチタニアナノ流体の熱伝導率を調査しました。彼らは、TiO 2 の熱伝導率を観察しました。 彼らが準備したナノ流体は、分散剤の含有量が高いため、従来のモデル予測よりもわずかに低かった。そして、結果は、TiO 2 ナノ流体は、他のレポートに示されているように、パイプフローで異常な熱伝導率の向上や対流熱伝達係数を示しません。
パーティクルシェイプエフェクト
ナノ粒子の形状とサイズの影響は、粒子負荷の影響ほど広くは調査されていません。既存の研究では、粒子の形状やサイズがTiO 2 の熱伝導率に大きな影響を与えることは示されていません。 ナノ流体。これはおそらく、この効果の量が比較的少ないためです。 Murshed etal。 [62] 2種類のTiO 2 を分散 分散剤としてCTABを使用したナノ粒子水。 1つのタイプは、直径10nm×40nmの棒状です。もう1つのタイプは、直径15nmの球形です。彼らは、両方の種類のTiO 2 の熱伝導率を観察しました。 ナノ流体は粒子負荷の増加とともに増加しましたが、棒状粒子は球状粒子よりも多くの寄与がありました。前者と後者の熱伝導率の最大の向上は、それぞれ約33%と30%でした。 Chen etal。 [64]は、TiO 2 で直交して作られた4種類のナノ流体の有効熱伝導率を研究しました。 ナノ粒子(25 nm)およびTiO 2 ナノチューブ(10nm×100nm)、それぞれ水とEGをベース流体として使用。彼らは、TiO 2 の強化の違いを発見しました。 ナノ粒子とTiO 2 熱伝導率のナノチューブは大きくありませんでしたが、強化はハミルトン-クロッサー方程式の計算値よりもはるかに大きくなっています。
温度効果
温度は、TiO 2 の熱伝導率に影響を与えるもう1つの重要な要素です。 ナノ流体。図2は、TiO 2 の熱伝導率の向上に対する温度の影響を示しています。 さまざまな研究におけるナノ流体。王ら。 [67]は、水ベースのTiO 2 の熱伝導率に対する粒子の負荷と温度の影響を調査しました。 ナノ流体。結果は、作動温度がより重要な正の役割を果たし、より高い温度での熱伝導率により多くの貢献をすることを示しました。彼らはまた、結果が温度依存のブラウン運動と微小対流を考慮して決定された理論値と一致したと結論付けました。 Reddy etal。 [68] TiO 2 の熱伝導率を調査しました さまざまな温度で0.2〜1.0%の範囲のさまざまな粒子負荷のナノ流体。そして彼らは、TiO 2 の熱伝導率を観察しました。 ナノ流体は、粒子の負荷と温度の両方の増加とともに増加します。ヤンら。 [58] TiO 2 を追加 ナノ粒子をアンモニア水に変換して、二成分流体ベースのナノ流体を調製します。彼らはまた、温度の上昇が二元TiO 2 の熱伝導率の増加をもたらす可能性があることを発見しました。 ナノ流体からベース流体へ。
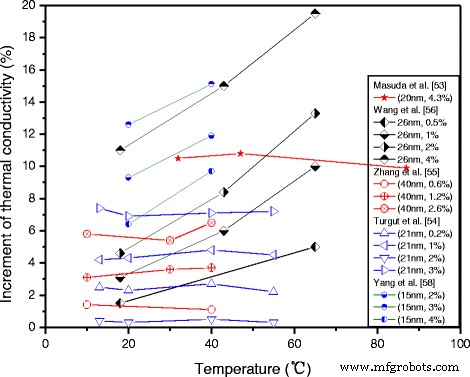
TiO 2 の熱伝導率の向上に対する温度の影響 さまざまな研究におけるナノ流体
上記の結果は、TiO 2 ナノ粒子は、TiO 2 の熱伝導率により多くの貢献をすることができます 高温でのナノ流体。ただし、温度の影響に関するいくつかの特異な結果も含めることができます。 Turgut etal。 [54]は、脱イオン水ベースのTiO 2 の有効熱伝導率を調査しました 13、23、40、55°Cの温度でのナノ流体。彼らは、粒子負荷の増加とともに熱伝導率が増加することを観察しましたが、温度の変化はTiO 2 の有効熱伝導率にほとんど影響を与えません。 ナノ流体。さらに、いくつかの結果は、温度が有効熱伝導率に影響を与えることを示しました。 DuangthongsukとWongwises [69]はTiO 2 を中断しました 体積負荷範囲が0.2〜2%の水中のナノ粒子であり、15〜35°Cの温度範囲でデータを収集しました。彼らは、TiO 2 の測定された熱伝導率を観察しました。 –水ナノ流体は、粒子の負荷と温度の両方の増加とともに増加しましたが、温度が上昇すると熱伝導率は減少しました。彼らはその理由をベース流体の熱伝導率のより速い成長速度に帰した。
TiO 2 の熱伝導率に対する温度の役割が不確かな理由 ナノ流体は、ナノ流体の熱伝導率の複雑なメカニズムに起因する可能性があります。温度が変化すると、構造、表面活性、安定性、粒子の特性、分散剤の特性などの他のパラメータが変化する可能性があり、これらのパラメータは一般に作業ごとに大きく異なります。したがって、TiO 2 の熱伝導率に対する温度の影響 ナノ流体は、特定のナノ粒子とベース流体のタイプに関連しています。この観察結果は、アナターゼ型およびルチル型TiO 2 について、温度依存の熱伝導率の挙動が研究されたCabaleiro etal。の研究[41]によってさらに改善できます。 ベース流体としてそれぞれエチレンとプロピレングリコールを含むナノ流体。これら4種類のTiO 2 の熱伝導率の温度依存性 ナノ流体を図3に示します。4種類のナノ流体すべてが、対応するベース流体よりも高い熱伝導率を示したことがわかります。 TiO 2 では温度がさまざまな役割を果たしました 異なるナノ結晶構造のナノ粒子を含み、異なるベース流体を含むナノ流体。熱伝導率は、EGベースのナノ流体の温度が上昇するにつれて増加し、研究範囲の温度によって最大11.4%増加しましたが、PGベースのナノ流体の温度とはほとんど無関係であるように見えました。
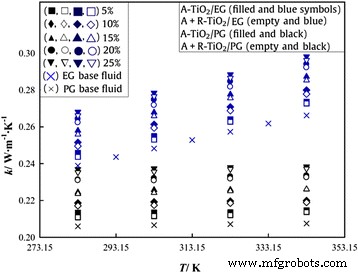
4種類のTiO 2 の熱伝導率の温度依存性 ナノ流体[41]。エルゼビアの許可を得て複製
基本流体効果
ベース流体の成分も、TiO 2 の熱伝導率に影響を与える可能性があります ナノ流体。 Chen etal。 [64]球形および管状のTiO 2 の有効熱伝導率を測定しました それぞれベース流体として水とエチレングリコールを使用したナノ流体。彼らは、TiO 2 の両方の強化が ナノ粒子とTiO 2 EGをベース流体として使用したナノチューブは、水をベース流体として使用したナノチューブよりも高かった。 Reddy etal。 [68]は、水ベース、EG / W(40%:60%)ベース、およびEG / W(50%:50%)ベースのTiO 2 の熱伝導率の向上を発見しました。 ナノ流体は、TiO 2 の体積濃度の場合、それぞれ0.649から5.01%、1.94から4.38%、および10.64から14.2%に増加しました。 ナノ粒子は、室温(30°C)で0.2%から1.0%に増加しました。しかし、いくつかの反対の結果も観察することができます、Cabaleiro等。 [41]は、TiO 2 の熱伝導率の向上を発見しました。 EG、PG、またはパラフィンオイルをベース流体として使用したナノ流体は、水をベース流体として使用したナノ流体よりも明らかに低かった。また、Sonawane et al。のレポート[70]では、TiO 2 の熱伝導率のため、ベース流体の効果は複雑でアクセスできないと考えられていました。 粒子負荷が1vol。%のナノ流体は、パラフィンオイルベースのナノ流体>水ベースのナノ流体> EGベースのナノ流体のシーケンスに従い、純粋なベース流体のナノ流体は、水> EG>パラフィンオイルのシーケンスに従いました。彼らは、粘度効果の観点からこの不規則な観察を分析し、ベース流体の粘度が低いほど、ナノ流体の熱伝導率の向上にさらに貢献できると考えました。
界面活性剤効果
界面活性剤の添加は、TiO 2 の熱伝導率に対するもう1つの重要な要素です。 ナノ流体。いくつかの結果は、界面活性剤が熱伝導率にプラスの影響を与えることを示しました。 Saleh et al。の[65]は、TiO 2 の熱伝導率に対するさまざまなタイプの界面活性剤の影響を研究しました。 –水ナノ流体、および結果を図4に示します。3種類の界面活性剤のすべてがナノ流体の熱伝導率を大幅に改善する可能性があり、安定剤としてSDSを使用したナノ流体が最大の増強を示し、次にスタビライザーとしてCTABとSpan-80。そして彼らは、粒子の分散安定性と表面特性がナノ流体の熱伝導の強化に関与していると考えました。
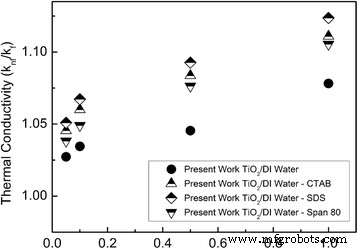
TiO 2 の熱伝導率に対するさまざまな界面活性剤の影響 –水ナノ流体[65]。エルゼビアの許可を得て複製
界面活性剤の効果についてもいくつかの異なる結果があります。ヤンら。 [58]は、ベース流体中のアンモニアの含有量が増加すると、TiO 2 の熱伝導率比が増加することを発見しました。 TiO 2 の安定性により、ナノ流体も増加します アンモニア水ナノ流体は、より高いpH値で改善されます。また、低濃度の界面活性剤PEG1000およびPAAは、粒子やアンモニア含有量、温度などの熱伝導率に対する他のインパクトファクターよりも影響が比較的小さくなります。ただし、PEG1000は安定性を向上させることができますTiO 2 ナノ流体の熱伝導率の改善を誘発するアンモニア-水ナノ流体。 Murshed etal。 [62]は、オレイン酸とCTABがTiO 2 の分散安定性を改善できることを発見しました。 実験で使用された界面活性剤含有量が非常に低かったため、ナノ流体の熱物性および単相熱伝達係数に影響を与えないナノ流体。 0.01〜0.02 vol。%。界面活性剤が抑制効果を持っていることを示したいくつかの結果もあります。 Utomo etal。 [66]は水ベースのAl 2 の熱伝導率を調査しました O 3 およびTiO 2 ナノ流体。彼らは、安定剤の高負荷により、これら2種類のナノ流体の有効熱伝導率が低下する可能性があることを発見しました。
超音波処理効果
超音波処理は、TiO 2 の熱伝導率にもいくつかの影響を示しました。 ナノ流体。 Ismay etal。 [71]は、TiO 2 の熱伝導率を発見しました。 –水ナノ流体は、pH値が7に近いときに最大に達し、2時間の超音波処理によってさらに改善されました。そして彼らは、凝集がパーコレーション効果による観察された増強を説明できると考えました。ソナワネ他[70]は、超音波時間による熱伝導率への影響について特定の研究を行い、その結果を図5a〜cに示します。さまざまな濃度の3種類のナノ流体すべてに見られ、熱伝導率の増加率は最初に増加し、次に超音波時間が増加するにつれて減少し、最大の増加は超音波処理時間60分で発生しました。その理由は次のとおりです。60分の最適な超音波処理時間により、ナノ粒子のブラウン運動と粒子とバルク液体間の分子間相互作用が強まり、熱伝導率が向上します。ただし、60分を超える長時間の超音波処理は、ナノ粒子のクラスター化と凝集を引き起こす可能性があります。これは、ナノ粒子の熱輸送と熱伝導率の低下の原因であると考えられていました。
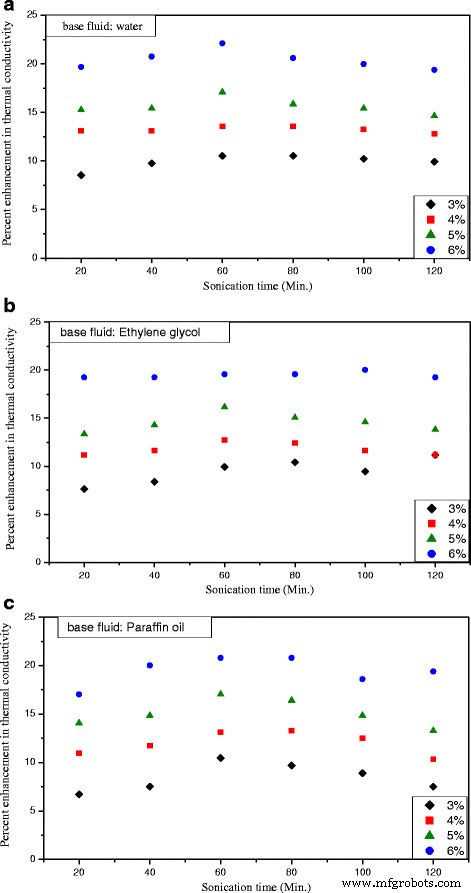
超音波処理時間の関数としての熱伝導率のパーセント向上。 a ベース液:水。 b ベース液:エチレングリコール。 c ベース液:パラフィンオイル。参考文献[70]
の実験データに基づいて再描画理論的研究
ナノ流体の理論的研究は、ナノ流体の分野における研究のホットスポットの1つです。近年、多くの熱伝導率モデルが提案されています。一般的に、ほとんどの従来のモデルはTiO 2 に使用できると考えられています。 特別な制限がない限り、ナノ流体。ただし、TiO 2 の熱伝導率の実験データには大きな違いがあるため、 ナノ流体の場合、単一のモデルがすべての異なる結果に適合することはほとんど不可能です。従来のモデルは個々のケースに適用するのが難しいため、TiO 2 の一部のターゲット熱伝導率モデル 近年、ナノ流体も提案されています。表1に、TiO 2 に特化した利用可能な熱伝導率モデルの方程式の概要を示します。 ナノ流体。一部のモデルでは、界面層[59、72]、ブラウン運動[73、74]、粒子サイズとアスペクト比[72、75]、凝集[76]などの要因が考慮されていることがわかります。また、実験的なフィッティングまたは分散分析によって提案されたモデルもいくつかあります[68、74、77、78、79、80、81、82]。これらのモデルは、個々のケースにのみ適していると結論付けることができます。ナノ流体の熱伝導率に関する理論的研究は多くの開発がなされてきましたが、最も根本的な欠陥は、さまざまな実験結果の大きな違いにあります。粒子のナノ構造と微動を定量的に説明することは難しいため、ナノ流体の熱伝導プロセスを包括的かつ正確に把握することはかなり困難です。したがって、個々のアプリケーションケースのモデルの精度が低いため、アプリケーションシステムを設計するためのナノ流体の熱伝導率を取得する最良の方法は、予備実験を実行することです。
<図> 図>上記の分析は、現在、TiO 2 の熱伝導率に影響を与える要因について論争と矛盾がまだ存在することを明らかにしています。 ナノ流体。粒子の負荷はナノ流体の熱伝導率と正の相関を示していますが、粒子の形状、サイズ、ベース流体の種類、温度、界面活性剤、超音波処理などの他の要因の影響は統一されています。粒子負荷効果の場合でも、熱伝導率の成長強度はサンプルごとに大きく異なります。さまざまな研究におけるナノ流体の熱伝導率の不一致は、主に、熱伝導率が多くの要因、特に定量分析や測定ではかなり難しい粒子のクラスター化や微動などのいくつかの微視的パラメータによって同時に影響を受けるためです。
別の論争は、ナノ流体の熱伝導の強化のメカニズムです。粒子のクラスター化と収集は、ナノ流体の熱伝導の向上に関与していると考えられています[48、50、51]。しかし、適切な界面活性剤または超音波処理による凝集が少ない安定したナノ流体も、より高い熱伝導率を示しています[62、65、66、70、71]。ナノ流体の熱伝導を高める主なメカニズムは、粒子のクラスター化や微動、またはその他のいくつかの要因をさらに分析する必要があります。
吸光度
再生可能エネルギーのクリーンな供給源として、太陽エネルギーは環境への影響を最小限に抑えます。しかし、太陽熱集熱器の開発は、従来の作動油の不十分な吸収特性によって制限されています。そのため、近年、ナノ流体技術がソーラーコレクターに徐々に使用され、優れた熱的および光学的特性を生み出しています。この新世代の熱伝達および太陽吸収流体は、太陽エネルギーの使用効率を向上させることが期待されています。
図6に示すように、ナノ流体ベースの集光型太陽熱温水暖房システムの典型的な概略図は、Khullar etal。のレポート[83]で見ることができます。彼らは、ナノ流体ベースの集光型ソーラーコレクターを実装することで、大幅な排出削減とエネルギー節約を達成できると考えました。チャジ他[84]は、TiO 2 を使用した小規模平板コレクターの効率に対する粒子の含有量と液体の流量の影響を調査しました。 ナノ流体。彼らは、TiO 2 を使用してコレクター効率の指標を見つけました ナノ流体は、欧州規格EN12975-2に基づくベース流体と比較して2.6〜7%増加しました。サイードら。 [85]使用済みTiO 2 –h 2 平板ソーラーコレクターの性能を高めるための作動油としてのOナノ流体。彼らは、調製されたナノ流体が1か月以上安定した状態を保つことができることを観察しました。その結果、水ベースの流体と比較して、エネルギー効率は76.6 vol。%の負荷と0.5 kg / minの流量で向上し、これらの動作条件で最高のエクセルギー効率16.9%を達成できることが示されました。
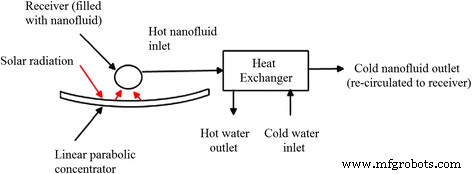
ナノ流体ベースの集光型太陽熱温水暖房システムの概略図。参照に基づいて再描画[83]
近年、ナノ流体を用いたソーラーコレクターの性能に関する理論的研究も展開されています。アリムら。 [86]は、Al 2 のエントロピー生成、熱伝達特性、および圧力損失を理論的に研究しました。 O 3 、CuO、SiO 2 、およびTiO 2 層流下の平板ソーラーコレクター内のナノ流体。彼らは、摩擦係数が水ベースの流体の摩擦係数とほぼ同じである一方で、あらゆる種類のナノ流体が性能を向上させることができることを発見しました。 Faizal etal。 [87]はまた、ソーラーコレクターにおけるこれらの4種類のナノ流体の性能に関する数値研究を実施しました。彼らは、4種類すべてのナノ流体のエネルギー節約が20%を超える可能性があり、その結果、温室効果ガスの排出量が削減されることを観察しました。
ソーラーコレクターのナノ流体による性能の向上は、一般に、熱伝達特性の向上と光学特性という2つの主要な要因に起因します。したがって、太陽吸収システムにおけるナノ流体の光学的性質も研究者によって調査された。サイードら。 [88]は、TiO 2 の日射吸収性能に関する実験と分析の両方の研究を実施しました。 およびAl 2 O 3 ナノ流体。彼らは、感光性の調査に0.1〜0.3 vol。%の2つの体積分率を使用しました。 Rayleigh、Maxwell–Garnett、Lambert–Beerのアプローチを含むいくつかの古典的な理論が、分析分析に採用されました。彼らは、TiO 2 の光学特性を結論付けました。 ナノ流体はAl 2 よりも高かった O 3 すべての粒子負荷に対して可視光の範囲内のナノ流体。彼等。 [89] TiO 2 の光-熱変換効率を比較しました –日当たりの良い条件と曇りの条件の両方での真空管ソーラーコレクター内の–水およびCNT–水ナノ流体。彼らは、CNT-水ナノ流体の温度上昇がTiO 2 のそれよりも高いことを観察しました。 –水ナノ流体。前者の光熱変換特性が後者よりも優れていることを示しています。
サイードら。 [90]ほとんどの研究は、ナノ流体の基本的な熱物理的および光学的特性に焦点を合わせていると考えた。粒子サイズ、形状、含有量、ベース流体の種類など、散乱と吸収の重要な要素に関する研究はほとんど見つかりませんでした。これらの要因を調べるために、彼らは関連する調査を実施し、20 nm未満では粒子サイズがほとんど影響を及ぼさず、粒子含有量が吸光係数に正比例することを観察しました。 20 nm TiO 2 を含むナノ流体の場合 ナノ粒子の場合、透過率は200〜300 nmの範囲の波長ではほぼゼロでしたが、400 nmでは71%、900 nmでは88%でした。彼らはまた、TiO 2 の体積分率を示唆しました。 ナノ粒子は0.1%未満である必要があります。これにより、はるかに優れた結果を得ることができます。
キムら[91] MWCNT、Al 2 を使用して詳細な理論的研究を実施しました O 3 、CuO、SiO 2 、およびTiO 2 高温U字管ソーラーコレクター内のPG(プロピレングリコール)-水(20:80)ベース流体を含むナノ流体。彼らは、ソーラーコレクター効率のコレクター効率は、MWCNT、CuO、Al 2 の順になっているため、追加されたナノ粒子の熱伝導率と明確に正の相関があることを観察しました。 O 3 、TiO 2 、およびSiO 2 ナノ流体。また、CO 2 の排出削減量も分析しました。 およびSO 2 だけでなく、世界中の電力とエネルギーの消費量。彼らの結果は、ナノ流体がエネルギー節約と排出削減に大きな可能性を秘めていることを裏付けています。それらの理論的結果は、さまざまなナノ流体の分散状況を考慮していないため、実際の性能を実験的に検証する必要があります。
偶然にも、異なるナノ流体を使用した平板ソーラーコレクターの同様の実験的研究がVermaらによって実施されました。 [92]。実験結果は、わずか0.75%の粒子体積負荷と0.025 kg / sの流量で、水と比較したナノ流体のエクセルギー効率が、グラフェンでそれぞれ29.32、21.46、16.67、10.86、6.97、5.74%増加することを示しました。 、CuO、Al 2 O 3 、TiO 2 、およびSiO 2 ナノ流体。また、エントロピー生成の低下はこのシーケンスに従いました。彼らの結果は、ソーラーコレクターの効率が、添加されたナノ粒子の熱伝導率と正の相関関係があることも裏付けています。
ただし、異なる結果をもたらすいくつかの研究もあります。マヒアンら。 [93]は、Cu、Al 2 を含む4つの異なる水ナノ流体を使用して、ミニチャネルベースのソーラーコレクターの性能を分析しました。 O 3 、TiO 2 、およびSiO 2 それぞれナノ粒子。彼らの結果は、Al 2 を示しました O 3 ナノ流体は最高の熱伝達係数を示し、最低の値はSiO 2 に属していました。 –水ナノ流体、ただし出口温度は次の順序に従いました:Cu> TiO 2 > Al 2 O 3 > SiO 2 ナノ流体。彼らはまた、TiO 2 のエントロピー生成を観察しました。 –水はAl 2 よりも低い O 3 –前者の熱伝導率にもかかわらず、水ナノ流体は後者よりも低くなります。
冷蔵
ナノ冷媒は、ナノ粒子と冷媒、および可能性のある潤滑剤で構成される特殊な種類のナノ流体です。 Nano-refrigerant is a new generation of refrigerant for using compression or absorption refrigeration, air conditioning systems, heat pumps, etc. In recent years, many studies regarding nano-refrigerants have shown that adding nanoparticles into refrigerants or lubricant can achieve a better system performance and energy efficiency.
Table 2 shows summary of related studies on TiO2 nanoparticle-based nano-refrigerants. It can be seen that the TiO2 nanoparticles can work normally and safely with many kinds of refrigerants, including R134a, R600a, R436a, R436b, R141b, R123, R12, R22, and R410a. It can be seen that most results showed that adding TiO2 nanoparticles could bring benefits to the refrigeration system and the lubricating oil system, such as improving the performance [94], reducing the energy consumption [95,96,97], and the irreversibility [98]. Also, some research focused on the heat transfer [99, 100] and pressure drop [101] of the nano-refrigerant system to investigate the effect mechanism of the nanoparticles. Li etal。 [102] investigated the coefficient of performance (COP) of the refrigeration system for both the cooling cycle and heating cycle, and the results showed that adding TiO2 nanoparticle would lead to a slight decrease in COP of the cooling cycle but a significant increase in COP of the heating cycle based on the power consumption of the compression. Bi et al. [96] experimentally investigated the reliability and performance of a domestic refrigerator with HFC134a as refrigerant and Mineral oil with TiO2 nanoparticles mixtures as lubricant. As illustrated in Fig. 7, the system main consists of fresh food storage room and frozen food storage room as well as refrigeration system pipelines. The results showed that the system TiO2 nanoparticles works normally and safely and adding 0.1 wt.% TiO2 nanoparticles can reduce 26.1% energy consumption while particle type has little effect on performance.
<図> 図>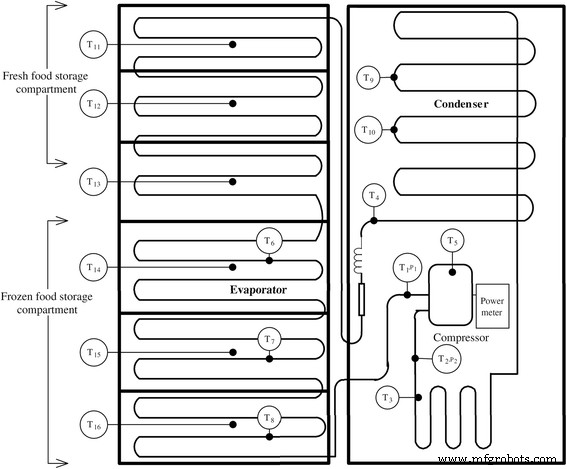
Schematic diagram of a domestic refrigerator with HFC134a, mineral oil and TiO2 nanoparticles [96]. Reproduced with permission from Elsevier
In addition, there is likewise a forward-looking study on the effect on the environment. Javadi and Saidur [103] observed that adding 0.1% of TiO2 nanoparticles to mineral oil-R134a could result in the maximum energy savings of 25% and reduce the CO2 emission by 7 million tons by year of 2030 in Malaysia.
It can be seen from Table 2 that the amounts of nanoparticles used in refrigerants were very low as below 0.1% [94,95,96,97,98,99,100,101,102,103], which can prevent clogging by the possible sedimentation of nanoparticles. However, although all results seemed positive, the long-term performance of the refrigeration system using nano-refrigerants is a great challenge.
Lin et al. [15] investigated the suspending ratio of (0.1 to 1%) nanolubricant–refrigerant after continuous alternation processes of condensation and evaporation. The schematic diagram and photographic view of their experimental system is shown in Fig. 8. They found that the degradation ratio was 28 to 73% after 20 times’ alternate operations. Also, they found lower particle loading can reduce the degradation speed. It can be concluded that the longtime performance of nano-refrigerant system is the essential step for further application in nano-refrigeration system.
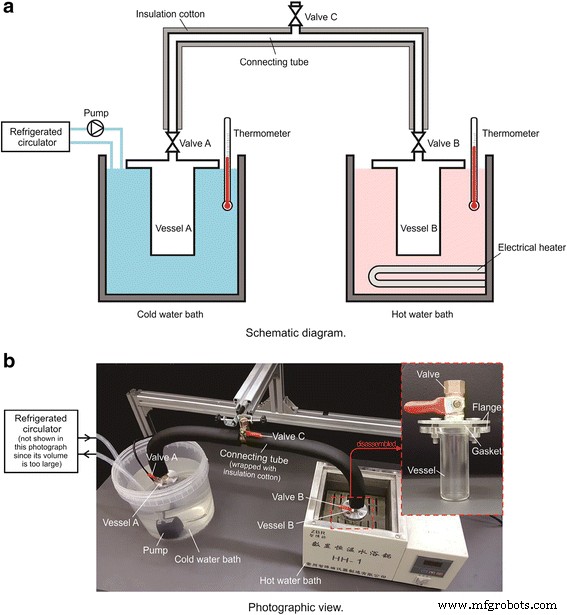
a 、 b Experimental setup for condensation–evaporation alternation [15]. Reproduced with permission from Elsevier
Energy Storage
The storage of latent heat is through the most efficient mean of storing thermal energy. The conventional PCMs have a shortcoming of inadequate heat transfer performance which can reduce the rate of storing and releasing thermal energy. Therefore, some researchers have studied the method of improving the heat transfer performance by adding nanoparticles into PCMs.
Usages of PCMs mainly include energy storage of heating or cooling capacity. Research on cool storage application of TiO2 nano-PCMs is relatively rare. Liu etal。 [104] find that thermal conductivity of saturated BaCl2 aqueous solution increases remarkably when adding a small amount of TiO2 ナノ粒子。 They found the thermal conductivity was increased by 15.65% as the TiO2 nanoparticle volume fraction was 1.13% at temperature of 15 °C. They thought this nanofluid is a good phase change materials (PCMs) with higher cool storage/supply capacity and rate compared with its base fluids, which exhibited good potential for being applied to cool storage as a substitute for conventional PCMs.
Another similar study was conducted by He et al. [105]. They also found the thermal conductivity of saturated BaCl2 aqueous solution can be distinctly enhanced by 12.76% when adding a small amount of TiO2 nanoparticles at −5 °C. Although decreases in the latent heat and specific heat and an increase in viscosity were found, those varieties have little effect on the cool storage system since the supercooling degree is reduced by 84.92%. They also thought that TiO2 -saturated BaCl2 aqueous solution is suitable for low-temperature energy storage industries.
Studies on cool storage of TiO2 nano-PCMs are in the minority, while most PCM applications focus on the heat storage. Table 3 shows a brief summary on the thermal conductivities and the latent heat of TiO2 nano-PCMs for thermal storage applications in existing literatures. Sharma et al. [106] prepared a composite of palmitic acid (PA) and TiO2 nanoparticles with SDBS as dispersant for thermal energy storage application. The preparation steps of PA–TiO2 composites are shown in Fig. 9. It can be observed that the dispersion methods including adding surfactant, stirring, and ultrasonic vibration were implemented under the condition that the temperature of the base PA is above the melting temperature to confirm its liquid state.
<図> 図>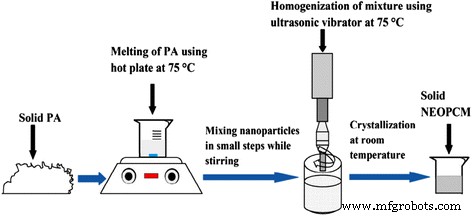
Preparation steps of PA–TiO2 composites [106]. Reproduced with permission from Elsevier
Their results showed that the thermal conductivity increased by 12.7, 20.6, 46.6, and 80% when the mass fractions of TiO2 nanoparticle were 0.5, 1, 3, and 5%, respectively. And they considered this PCM could be a good candidate as potential solar thermal energy storage materials due to its high latent heat and thermal reliability of palmitic acid. Harikrishnan et al. [107] dispersed TiO2 nanoparticles into PCM stearic acid and found this composite can accelerate the melting and solidification rates due to the enhanced heat transfer performance. They also observed that the addition of 0.3% nano-TiO2 nanoparticles can increase the thermal conductivity of stearic acid by approximately 63%. In their another research [108], they used stearic acid and lauric as base PCM and found an increment of 42% in thermal conductivity and a reduction of only 2% of latent heat of fusion. Motahar et al. [109] dispersed the TiO2 nanoparticles into organic PCM n-octadecane and found that the maximum enhancements of thermal conductivity in solid and liquid phases occurred at 3 and 4 wt.%, respectively. Moreover, the maximum average thermal conductivity enhancement for both phases was 26.6% when loading 5 wt.% nanoparticles.
Another experimental research focusing on the solidification process of PCM containing TiO2 nanoparticles was also performed by Motahar et al. [110]. They observed that the rheological behavior of liquid PCM–TiO2 at higher loading tends to Bingham fluids so that their solidification experiments were performed within 0–2.17 Bingham numbers. The results showed that the addition of TiO2 nanoparticles can enhance the thermal conduction process and hence increase the solidified volume. For particle mass loading of 1, 2, and 4%, the solidified volume fraction was increased by 7, 9, and 18%, respectively. At last, they proposed a universal correlation to predict the solidified volume fraction as a function of Fourier number, Rayleigh number, solid Stefan number, Bingham number, and particle loading.
Most of the results showed that when adding TiO2 nanoparticles, the thermal conductivity of PCMs can be greatly increased, while the latent heat will be decreased slightly, which is probably as a result of the thermal conductivity of nanoparticles which is much larger than the base composite, while the nanoparticles will not take part in the phase changing process as the base composite. However, in some case, both of the thermal conductivity and latent heat capacity of PCMs were considered to be elevated. Wang et al. [111] prepared nano-PCMs by adding TiO2 nanoparticles into paraffin. They found the addition of TiO2 nanoparticles can change the phase transition temperature and latent heat capacity of paraffin. The phase transition temperature dropped with <1% loading, while increased with>2% loading of particles. The latent heat increased firstly and then decreased as the loading of particles increase. And the turning concentration is 0.7 wt.%, at which a maximum latent heat capacity can be achieved. While the thermal conductivity of the nano-PCMs increased monotonously with the loading of TiO2 ナノ粒子。 When the loading of TiO2 nanoparticles reached 7 wt.%, the thermal conductivity was increased by 13% but the latent heat was reduced by 9%.
Heat Pipes
The characteristics of boiling heat transfer and critical heat flux enhancement of nanofluids can be utilized in the heat pipe to improve its performance and broaden the application range. And some numerical results [112, 113] have shown that for thermosyphon heat pipe, using the nanofluid could achieve a better heat transfer characteristics. Also, some researchers have carried out related research using TiO2 nanofluids.
Zhou et al. [114] tested gravity heat pipes filled with DI water and TiO2 nanofluids, where the concentration and filling ratio of nanoparticles were varied and the initial temperature distribution was given. The result indicated that the heat pipes filled with nanofluids had a lower start-up temperature and a shorter start-up time in evaporation section under the condition of a water bath. And the biggest temperature drop between the evaporation section and the condensation section for heat pipes filled with TiO2 nanofluids was lower than those filled with DI water. The start-up time of heat pipes with filling ratios ranged between 50 and 70% in the evaporation section increased with the increase of the filling ratio and heating temperature, but the small inclination angle had a negative effect on the start-up performance.
Saleh et al. [65] collected data from different nanofluid experiments, where particle volume loading was up to 1.0% and the temperature of measurements ranged from 10 to 60 °C. They discovered that these data agreed with the classical Brownian motion theoretical model. They also investigated experimentally the effect of nanofluids on the thermal performance of heat pipes by measuring the wall temperature and thermal resistance distributions between the evaporation and condensation section. They found that distilled water and nanofluids achieved the best heat transfer performance when the inclination was set to 45° and the charge volume ratio of working fluid was 60%.
In 2015, Monirimanesh et al. [115] designed a thermosyphon-type heat pipe heat exchanger (HPHX) using TiO2 nanofluids as the working fluid to save energy in an air conditioning system. Their experimental apparatus was constructed as shown in Fig. 10. They establish a pre-cooling and pre-heating device to produce altered conditions of the inlet air for investigating the performance of HPHX. The evaporator and condenser section of the HPHX functioned as a pre-cooler and reheating coil for the air conditioning system respectively. They also employed an electric heater and electric boiler to supply heat and steam into the entered fresh air by a fan for the purpose of simulating the hot and humid climate. Their results showed that using TiO2 nanofluids and increasing the HPHX number of rows could make a part of air condensed on the evaporator fin, which could enhance the energy in the pre-cooling section. The use of 3 wt.% TiO2 –methanol nanofluids in a four-row HPHX could achieve the highest energy savings ranging from 30.6 to 32.8% when the inlet air under the properties of 45 °C and 50–74% relative humidity. Based on a comprehensive consideration of the main purpose of supplying the energy required for reheating, they suggested that 2 wt.% TiO2 –methanol nanofluid for the four-row HPHX would have been adequate and more economical.
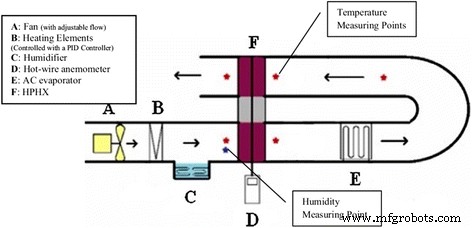
Schematic of the experimental apparatus [115]. Reproduced with permission from Springer
Mass Transfer
The mass transfer of nanofluids is another important application aspect of TiO2 nanofluids. Current research has shown that TiO2 nanofluids can be used to enhance the absorption process of CO2 and NH3 as well as the mass transfer coefficient of electrolyte fluids.
Li etal。 [116] prepared stable N -methyldiethanolamine (MDEA)-based nanofluids to strengthen the absorption performance of CO2 in the MDEA solution. The CO2 absorption characteristics in the gas/liquid interface of nanofluids were investigated by measuring the absolute pressure drop of gas. The mass fraction of MDEA was 50%. And they used two particle mass fractions of 0.1 and 0.4%. The results showed that the CO2 absorption rate increases with increasing temperature and it is enhanced by the added nanoparticles. However, at 20 and 30 °C, the enhancement caused by the mass fraction of nanoparticle (0.1 and 0.4%) reduced gradually. The effective absorption ratio varied from 1.03 to 1.14. Also, CO2 bubble absorption ratio increased with the increase of nanoparticle mass fraction.
Yang [117] prepared stable TiO2 nanofluids without adding dispersant and then carried out a comparative experiment on the falling film performance of absorption of ammonia gas by nanofluids and pure water. The schematic diagram of the experimental system for NH3 –H2 O nanofluid falling film absorption is shown in Fig. 11. They found that the absorption rate of ammonia gas can be increased by 10% when adding anatase TiO2 nanofluids. Wu [118] used the similar experimental device but changed the falling film tube of Fig. 11 into a fin tube. He investigated the effect of rutile TiO2 nanofluids on the ammonia absorption performance of falling film outside a fin tube. The result showed that the combined use of zigzag tubule and TiO2 nanofluids can strengthen the ammonia–water falling film absorption and the maximum increment can reach 60.8%.
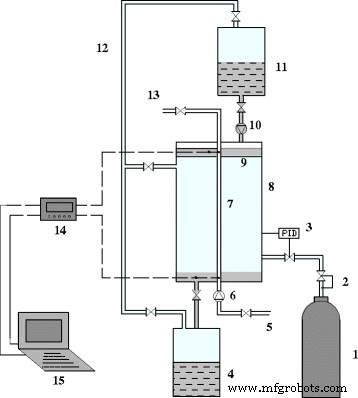
Schematic diagram of the experimental system for NH3 –H2 O nanofluid falling film absorption [117]. 1 NH3 vessel, 2 decompression valve, 3 constant pressure controller, 4 , 11 container of solution, 5 inlet of cooling water, 6 , 10 constant flow controller, 7 falling film tube, 8 visible absorber body, 9 solution distributor, 12 tubes for balancing pressure, 13 outlet of cooling water, 14 HP data acquisition instrument, 15 computer
Beiki et al. [119] investigated experimentally the turbulent mass transfer characteristics of TiO2 and γ-Al2 O 3 electrolyte nanofluids in a circular tube. The results showed that adding 0.015 vol.% TiO2 and 0.01 vol.% γ-Al2 O 3 could bring an increase in mass transfer coefficient of the electrolyte solution by 18 and 10%, respectively. They found that the enhancement ratio was independent of Reynolds number. The mass transfer coefficients increased firstly and then decreased as the nanoparticle loading increase. They attributed the cause of the existing of optimal particles’ loading to the clustering of nanoparticles and forming bigger agglomerates with smaller Brownian velocity when exceeding the optimum loading.
Coolant of Milling
As a coolant, nanofluids’ heat transfer enhancement characteristic can improve the cooling performance [120]. Moreover, when nanofluids are used for milling, another characteristic of nanofluids viz. enhancement in wear resistance can also play an important role in extending the lifetime of the milling tool.
Yogeswaran et al. [121] investigated experimentally the effects of coolant of TiO2 –EG nanofluid on the tool wear and workpiece temperature at the various milling conditions when used for milling a stainless steel AISI 304. The milling tool was made of a TiN-coated carbide insert. The results showed that comparing to pure base fluid, the workpiece temperature was reduced by 30% when using the nanofluid as coolant. The tool wear from milling using the EG-based TiO2 nano-coolant is much less than using the normal commercial coolant because the nanofluids can reduce the heat penetrating into the inserts. And the tool life is increased as a result of the nanoparticles reduces the damage on the edge of the tool.
Muthusamy et al. [122] also compared the efficiency of nanoparticle-based coolant (TiO2 –EG) and conventional water-soluble coolant on the tool life and wear performance of a TiN-coated carbide insert in the end-milling process of AISI304 stainless steel. The results showed that using TiO2 –EG nanofluid as coolant could increase the tool life from 32.67 to 54.9 min (increased by 40.55%) comparing to that using TiO2 –EG nanofluid as coolant instead of water-soluble coolant. They attributed the cause to a Ti nanoparticle layer on the edge of the insert formed during the milling process when using TiO2 –EG nanofluid, which can be proved from the SEM and EDX of cutting edge as shown in Fig. 12. When using nanofluids as coolant, the oxidation still occurred despite the cutting temperature was reduced at the interface of the tool and workpiece since it can be found from Fig. 12 the O peak on the EDX spectrum. The hard oxidation layer was formed due to the entering of oxygen from TiO2 –EG nanofluid into the tool–workpiece interface. Then, the hard oxidation layer can protect the tool from micro-cracking and chipping wear because it could not be easily detached despite under the severe impact of the milling force and took parts of the tool surface from the workpiece.
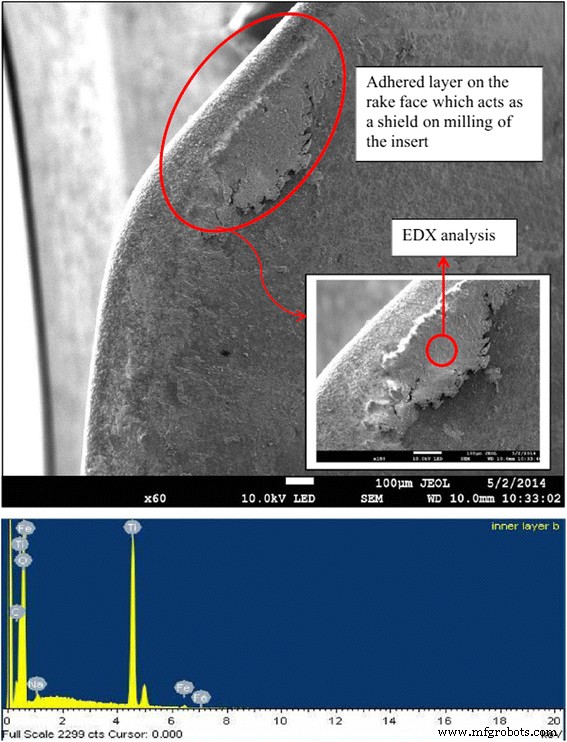
SEM and EDX of cutting edge [122]. At a cutting speed of 1500 rpm, feed rate of 0.02-mm tooth, and axial depth of 0.1 mm using nanoparticle-based coolant at a cutting distance of 180 mm (×60 magnification). Reproduced with permission from Springer
Challenges and Future Works
Challenges
The above energy-related examples have exhibited the extensive application prospect and excellent properties of TiO2 nanofluids. Although in some cases, especially in heat transfer applications, the heat transfer performance of TiO2 nanofluids are not better than that of Ag, Cu, and CNT nanofluids, TiO2 nanofluid is also a good choice due to their comprehensive properties for instance better dispersion and chemical stability, security, and economy.
Although TiO2 nanofluids have showed great enhancement in heat transfer of solar collectors, refrigeration, energy storage, heat pipes, and coolant of milling, the investigations on the performances including dispersion stability and heat transfer performance after running operations are in great lack. Most dispersion stability studies are in static conditions, but it is important that the nanofluids prepared should be treated in the practical application conditions to examine the dynamic cycle stability and the sustainability of both system performance and components of nanofluids.
Generally, the biggest downside in application of nanofluids is the sedimentation and degeneration of nanoparticles after long running which makes the long-term performances of nanofluid system challenged. Some researchers have proposed a new idea and a novel method to re-disperse the aggregates in real time of the running system [123]. However, the concrete effect of the device has to be verified experimentally, and then, the design and location of the re-dispersion device needs to be improved. The surfactant is expected to have positive effect on the re-dispersion characteristic of aggregates. However, one of the biggest flaws in using surfactants is the occurrence of foaming when the fluids are under flowing or heating conditions which would have adverse impacts on the heat or mass transfer application of nanofluids. This defect suggests the amount of surfactant employed in the nanofluids should be limited.
Another great limitation in application of nanofluid is the increase in pumping power and pressure drop of nanofluids, which is essential for the high-quality application of solar collectors, refrigeration, and heat pipes. For instance, Sajadi and Kazemi [124] found the proportional increase in pressure drop of TiO2 nanofluids is higher than that of heat transfer coefficient. While Teng et al. [125] found the pressure drop proportion of TiO2 nanofluids for turbulent flow is lower than that for laminar flow. Therefore, if the extra energy consumption by the increased viscosity of nanofluids exceeds the benefit from the heat or mass transfer enhancement, there will be no application prospect. The most extreme case is when a large amount of agglomerations emerge, the pumping power and pressure drop of nanofluids will be greatly increased, which might lead to serious impact on system performance. Moreover, based on the similarity principle in heat transfer study, for instance in forced convection process, Nusselt number is determined by Reynolds number and Prandtl number, different thermal conductivity and viscosity will induce different Nu even though for the same experimental heat transfer coefficient. Therefore, the properties of nanofluids are essential for quantitative study in those application fields.
Future Works
As a widely used material in considerable fields, TiO2 has been explored several hundred years, and its nanofluidic form is also firmly worth studying and expected to make greater contributions owing to the outstanding physical and chemical properties. This paper provides a summary of the research outcomes of TiO2 nanofluids up to now, including the preparation and stability of TiO2 as well as three vital properties of TiO2 nanofluids. It can be concluded that TiO2 nanofluids show very comprehensive applications in heat transfer or other energy fields due to their good dispersion stability in both hydrophilic and lipophilic liquids, nontoxic and non-corrosive natures, chemical stability, lower price, and good appearance. Therefore, TiO2 nanofluid is thought as one of the closest kinds to practical industrial application environment because of their better dispersion and chemical stability, security, and economy.
However, although TiO2 nanofluids have shown enormously exciting potential applications, before commercialization of nanofluids, some urgent problems are summarized as follows:
Firstly, acquiring high-quantity nanofluid with outstanding long-term and high-temperature stability is the fundamental of the entire research since in any practical application, it is essential to have a stable suspension.
Secondly, the way to enhance and keep the stability of nanofluids in real time is a key issue in the actual use since the sedimentations of nanoparticles seem inevitable after a long-term running. The method to re-disperse the aggregation of nanoparticles in real time by adding some dispersion device in the system with functions of ultrasound or agitation might be a useful option [123].
Thirdly, although the surfactants were used to improve the dispersion and adhesion performance of nanoparticles in liquid, the effect of surfactants on the physical properties and system performance needs to be investigated. The amount of surfactants should be investigated experimentally owing to the positive and negative effects of surfactants.
Fourth, the pumping power or pressure drop of nanofluids is another challenge for the engineering application. Using nanofluids with higher viscosity than base fluids will induce a higher pressure drop and hence needs more pumping power [125]. The method to achieve higher heat transfer coefficient and lower pressure drop needs to be further studied.
Fifth, the waste management of the invalid nanofluids should also be considered when applying them to industrial systems. The impact on the environment by the nanofluids restricts many kinds of nanofluids containing heavy metal, toxic substance, or other hazardous substances. The super whiteness dyeing behavior of TiO2 nanofluids should also be noticed to prevent the environment getting contaminated.
Sixth, although some studies have analyzed the entropy generation in tubes [126], microchannels [127], sheet, and other types of flow [128, 129], the entropy generation characteristic of nanofluid in the full system is actually the most important parameter for the full-system application or designing.
Last but not least, there is lack of evaluation index on the performance of nanofluids, especially on the stability, adhesion, and property sustainability of nanofluids. There is no unified indicator to evaluate the stability and adhesion of nanofluids. The uniform evaluation indexes on the different properties of nanofluids are needed [130].
The above problems are urgently needed to solve for the further application of TiO2 nanofluids, which point out the directions of the future works in this field. It is believed that these problems and challenges will be solved or reduced with the development of nanofluid technology in the future.
Conclusions
This second part of the review summarizes recent research on application of TiO2 nanofluids and identifies the challenges and opportunities for the further exploration of TiO2 nanofluids. It can be concluded that although particle loading has exhibited a positive correlation with thermal conductivity of nanofluids, the effects of other factors including particle shape, size, base fluid type, temperature, surfactant, and sonication are unified. Even for particle loading effect, the intensities of growth in thermal conductivity differ widely for different samples. TiO 2 nanofluids have shown good applications in many energy-related filed. However, the indeterminacy of long-term performances for both nanofluid and system and the increment in pressure drop are needed to investigate for further application. The forecast research hotspots are regarded as the long-term and high-temperature stability and re-disperse the aggregation of nanoparticles in real-time system, the required amount of surfactants, the heat transfer and pumping power characteristics, and the evaluation index on the stability, adhesion, and property sustainability of nanofluids.
ナノマテリアル