CuおよびCu / Cu2Oナノ粒子とCuSO4を使用したポリ(ブチレンアジペート-コテレフタレート)に基づくナノコンポジットとコンポジットの抗菌効果の比較研究
要約
ナノコンポジットおよびポリ(ブチレンアジペート-コテレフタレート)(PBAT)に基づくコンポジットは、市販の銅ナノ粒子(Cu-NP)、銅/酸化第一銅ナノ粒子(Cu | Cu 2 )を使用して合成されました。 O-NPs)、および硫酸銅(CuSO 4 )、 それぞれ。 Cu | Cu 2 O-NPは、化学還元を使用して合成され、X線回折(XRD)および透過型電子顕微鏡(TEM)によって特徴付けられました。 Cu | Cu 2 の合成 O-NPはCuとCu 2 の混合物を生成しました O、直径約40nmの球形の金属CuとCu 2 直径150nmのO。ナノコンポジット(NC)とコンポジット材料(MC)を準備するには、NPとCuSO 4 塩は、ex situ法により、1、3、および5%p / pの濃度でPBATマトリックスに組み込まれました。フーリエ変換赤外分光法(FTIR)、引張試験、示差走査熱量測定(DSC)、熱重量分析(TGA)、および寒天拡散アッセイを、構造、熱機械、および抗菌の特性評価に使用しました。結果は、強化がPBATの化学構造を変更せず、結晶化の割合をわずかに増加させたことを示しました。 PBATの機械的特性と熱的特性は、それぞれ引張強度と熱安定性のわずかな増加を除いて、フィラーの添加によってあまり変化しませんでした。寒天拡散抗菌アッセイは、NCおよびMCが非耐性菌 Enterococcus faecalis に対して良好な阻害反応を示すことを示しました。 、ストレプトコッカスミュータンス 、および黄色ブドウ球菌 。 CuSO 4 に基づくMC 耐性菌 Acinetobacter baumannii に対しても、最高の殺生物効果を示しました。 。
はじめに
ほとんどのプラスチック材料は化石燃料から製造されており、実質的に非分解性であるため、経済的および環境的持続可能性に関する懸念が生じます[1、2]。したがって、別の供給源からの生分解性材料の開発と合成は、石油ベースのプラスチックの生産を減らすことを目的として、科学界から多くの注目を集めています[3,4,5]。生分解性ポリマーは、化石燃料の有望な選択肢として、ナノテクノロジーを通じてより優れた特性を持つようになったバイオナノコンポジットとして知られる新しいクラスの材料とともに、これらの問題を解決する上で基本的な役割を果たし始めています[6,7,8,9 、10]。
バイオナノコンポジットは、無機ナノ材料が分散した有機マトリックスで構成されています[8、11、12、13]。ナノ粒子、ナノチューブ、ナノシート、ナノワイヤー、ナノクレイなどの無機成分のさまざまな形態とサイズは、ポリマーマトリックスの特性にかなりの影響を及ぼします。表面積、高い表面反応性、優れた熱安定性、および無機成分とポリマーマトリックスの高い機械的強度の間の相乗効果により、光学的、熱的、機械的、磁気的、および光電子的特性が改善されます[14、15、16]。 。高分子化学およびマイクロおよびナノファブリケーション技術の幅広い革新により、ポリマーバイオナノコンポジットの研究が促進され、改良された構造の製造だけでなく、興味深い特性と高度な用途を備えた新しい機能性材料の調製も行われています[17,18 、19]。ポリ乳酸(PLA)[20]やポリ(ブチレンアジペート-コテレフタレート)(PBAT)などの天然または合成由来のいくつかの生体高分子が広く研究されています[21、22]。
ナノコンポジットのマトリックスとして現在使用されているポリマーの1つは、PBATです[23]。この合成生体高分子は、ポリマー鎖に含まれるモノマー1,4-ブタンジオール、アジピン酸、テレフタル酸をベースにした線状脂肪族生分解性ポリエステルです[24]。その特性は、高分子量と長鎖分岐分子構造のために低密度ポリエチレンの特性と似ており、柔軟性があります[24、25、26]。 PBATの主な制限は、機械的強度が低いことです。ただし、ナノサイズの負荷を追加することで、この欠点を克服できるため、この材料に、より優れた熱機械的特性などの多機能特性を与えることができます[6、27]。
現在、既知の抗菌活性を有するナノ粒子をポリマーマトリックスがすでに保有している抗菌特性に組み込むか、または増強することにより、微生物のコロニー形成を制御または防止できるバイオナノコンポジットを開発する緊急の必要性もあります。後者の場合、ポリマーマトリックスの殺生物能力の大幅な改善は、バイオナノコンポジットの2つの成分間の相乗効果に関連しています[28、29]。したがって、ポリマーはナノ粒子のサポートマトリックスを提供するだけでなく、抗菌性能を向上させ、バイオメディカルアプリケーションや気管内チューブや血管および尿道カテーテルなどの医療機器のさまざまな要件を満たすためにバイオナノコンポジットの可能なアプリケーションを拡張することもできます[30 、31,32]。ただし、医療機器でのPBATの使用は広く研究されていません。一部の臨床応用での使用の可能性を報告している記事はごくわずかです[1]。
いくつかの調査では、抗菌剤としての金属ナノ粒子の使用が報告されています。これらの材料の固有の生物学的特性は、関与する金属、粒子サイズ、構造、表面積などのいくつかの要因に依存します。これらの要因のすべての可能な組み合わせは、抗菌剤耐性を遅らせる可能性があります[33]。ナノコンポジットのほとんどの抗菌研究は食品包装に焦点を合わせており、殺生物活性は常に同じ細菌を対象としてきました。バクテリアが薬物と同じように殺生物性ナノ粒子に耐性を持つようになるかどうかは定かではありません。したがって、この作業の目的の1つは、歯科用器具の製造に使用できる可能性のある、さまざまな濃度のCu-NPを含むPBATを含むナノコンポジットの抗菌活性を評価することでした。さらに、PBATベースの材料の熱機械的および抗菌特性に関する完全な比較研究を実施しました。 PBATナノコンポジットは、3つの異なる濃度のCuナノ粒子を使用して調製されました。同様に、ナノコンポジットはCu | Cu 2 を使用して調製されました 負荷としてのO-NP。最後に、CuSO 4 ベースの複合材料は、ナノコンポジットの調製に使用されたのと同じ濃度で調製されました。ナノコンポジットとPBATコンポジットの殺生物活性を黄色ブドウ球菌に対して評価しました。 、毛嚢炎、癤炎、結膜炎などの皮膚感染症の原因です。 ストレプトコッカスミュータンス 、これは歯垢と歯科用バイオフィルムに部分的に関与しています。および Enterococcus faecalis および Acinetobacter baumannii 、特に病院環境で、人間を危険にさらす感染症を引き起こす可能性があります。
材料と方法
資料
ナノコンポジットの調製に使用されるPBAT(Ecoflex)は、BASF(Ludwigshafen、ドイツ)から提供されました。その分子構造は、追加ファイル1:図S1(補足資料)に示されています。 99.99%の純金属Cuナノ粒子(Sigma-Aldrich、セントルイス、ミズーリ州、米国)は、直径が100〜200nmでした。 Cu | Cu 2 の合成用 O-NP、CuSO 4 前駆体としてアスコルビン酸(C 6 H 8 O 6 )還元剤として、およびpHコントローラーとして水酸化ナトリウム(NaOH)。さらに、CuSO 4 (Sigma-Aldrich)を使用して複合材料を準備しました。
化学還元によるナノ粒子の合成
カーンらによって提案された合成方法。 [34]を使用してCu | Cu 2 を取得しました O-NP。合成はCuSO 4 を溶解することから始まりました ×5H 2 蒸留水中のOで、120mLの0.1M溶液を取得します。次に、120mLのCuSO 4 プロピレングリコール浴に浸したフラスコに加え、続いて50mLのC 6 を急速に加えた。 H 8 O 6 解決。温度を80℃に上げながら、混合物を約390rpmで30分間激しく撹拌し、その後、30mLのNaOH溶液を滴下し、溶液を2時間連続して撹拌した。最終溶液を一晩静置した後、上澄み液を除去した。濃縮物を遠心分離し、蒸留水およびエタノールで洗浄した。最後に、超音波装置を使用して粒子を分散させ、ペトリ皿に入れ、60°Cで一晩オーブン乾燥しました(追加ファイル1:図S2を参照)。
ナノコンポジット合成
ナノコンポジットと複合材料を準備するには、Cu-NP、Cu | Cu 2 O-NP、およびCuSO 4 塩は、1、3、および5%の濃度でPBATマトリックスに組み込まれました。まず、PBATを溶融し、次にNPを添加し、トルクレオメーター(モデル835205、Brabender GmbH&Co。KG、ドイツ、デュイスブルク)で60rpm、作業温度140°C(ドイツ、デュイスブルク)で7分間混合しました。追加ファイル1:図S4)。最大負荷は5%でした。これは、負荷が高いほどラマンスペクトルに蛍光効果が生じるためです(追加ファイル1:図S3)。
特性評価
得られたナノコンポジットとコンポジット材料は、PBATポリマーに関するそれらの違いを研究するために特徴付けられました。同様に、さまざまな濃度のCu-NP、Cu | Cu 2 O-NP、およびCuSO 4 ポリマーの内部は、その機械的、熱的、形態的、構造的、および殺菌性に影響を及ぼしました。
Cu-NPおよびCu | Cu 2 O-NPは、X線回折(XRD)および透過型電子顕微鏡(TEM)によって特徴づけられました。 Cu-NP(NCs-PBAT / Cu)およびCu | Cu 2 を含むPBATナノコンポジット O-NP(NC-PBAT / Cu | Cu 2 O)およびCuSO 4 を含むPBAT複合材料 (MC-PBAT / CuSO 4 )は、熱重量分析(TGA)、示差走査熱量測定(DSC)、走査型電子顕微鏡(SEM)、フーリエ変換赤外分光法(FTIR)、XRD、引張試験、および寒天拡散を使用した抗菌活性アッセイによって特徴づけられました。各ナノコンポジットの100mm×100mm×1mmの板状サンプルは、各分析で均質化されたサンプルが同じサイズになるように準備されました。プレート形状を取得するには、NCs-PBAT / Cu、NCs-PBAT / Cu | Cu 2 O、およびMC-PBAT / CuSO 4 Labtech油圧プレス(モデルLP-20B; Labtech Engineering Co.、Ltd.、Samutprakarn、Thailand)を使用して、160°C、110バールで5分間成形しました。予熱時間と冷却時間はそれぞれ15分と1分でした(追加ファイル1:図S4)。
形態学的および構造的特性
ナノ粒子のナノメートルスケールを検証し、合成された粉末がCuとCu 2 の混合物であることを確認する ナノ粒子については、XRDを使用して構造解析を実行し、TEMを使用して形態素解析を実行しました。
Cu | Cu 2 のTEM顕微鏡写真 O-NPは、JEM 1200 EX II透過型電子顕微鏡(JEOL、Ltd.、Tokyo、Japan)を使用して120kVの電圧で取得しました。サンプルは、エタノールで希釈したナノ粒子の液滴を200メッシュのカーボンコーティングされた銅グリッド上に配置することによって準備されました。さらに、ナノ粒子は電子線回折パターンによって分析されました。
Cu-NPのXRDスペクトル、Cu | Cu 2 O-NP、ナノコンポジット、および複合材料は、Bruker Endeavour回折計(モデルD4 / MAX-B; Bruker、米国マサチューセッツ州ビレリカ)を使用して取得しました。 2θの掃引は4から80°で、0.02°のステップと1秒のカウント時間でした。回折計は、銅陰極ランプ(λ)を使用して20mAおよび40kVで動作しました。 =1.541Å)。
ナノコンポジットのFTIRスペクトルは、減衰全反射(ATR)機能を備えたSpectrum Two FTIR分光計(×1720)(PerkinElmer、マサチューセッツ州ウォルサム、米国)を使用して取得しました。各スペクトルは、4000〜500 cm -1 の範囲での連続スキャンによって取得されました。 解像度は1cm -1 。
機械的特性(引張試験)
ASTM D638規格に基づく引張試験は、smarTensユニバーサル試験機(005モデル、Emmeram Karg Industrietechnik、ドイツ、クライリング)で、試験速度50mm / min、ロードセル1kNで実施されました。 Vタイプの試験片は、160°Cの成形温度で圧縮して製造されました。予熱、プレス、冷却時間はそれぞれ7、5、1分でした。調査中の各NCおよびMCの5つのサンプルを製造し、引張強度、極限伸び率、および弾性率を取得しました。
熱特性
TGAは、TG209FIIris®サーモマイクロバランス(NETZSCH-GerätebauGmbH、セルプ、ドイツ)を使用して実行されました。 3〜10 mgの範囲のサンプルをアルミニウムるつぼに入れ、機器にロードしました。温度の関数としての質量変化は、N 2 の下でサンプルを10°C /分の速度で20から600°Cに加熱することによって測定されました。 雰囲気。
DSC分析は、NETZSCH示差走査熱量計(DSC 204 F1モデル)を使用して実施された。ナノコンポジットサンプル(5〜10 mg)を密封されたアルミニウムるつぼに入れ、一定のN 2 の下で10°C /分の速度で25から200°Cに加熱しました。 20 mL / minの流量。融解温度( T m )はこのDSC分析から得られました。
寒天拡散を使用したNCおよびMCの抗菌活性アッセイ
ナノコンポジットおよびCu-NP、Cu | Cu 2 に基づく複合材料の抗菌活性 O-NP、およびCuSO 4 寒天中での拡散成長速度論法を使用して決定された。分析は、Jaramilloらのプロトコルに従って2段階で実施されました。 [35]。細菌の4つの菌株が使用されました:2つの臨床菌株、 A。バウマニ (ABA 538)病院内感染および Eから分離。フェカリス (6.4)口腔感染症、および2つの収集株 Sから。アウレウス (ATCC)および S。ミュータン (ATCC 25175)。
最初の段階は、抗菌活性の定性的評価で構成され、3つの負荷濃度を使用すると非常にコストがかかるため、ナノコンポジットと複合材料の3つの濃度のどれを使用して定量的テストを実行するかを選択して実験計画を減らします。評価テストの後、最良の接触阻害を示した負荷率のサンプルが選択されました。定性テストを実行するには、 A。バウマニ (ABA 538)、 E。フェカリス (6.4)、 S。アウレウス (ATCC)、および S。ミュータン (ATCC 25175)をトリプチケースソイ寒天培地(TSA)に別々に播種し、37℃で一晩インキュベートしました。培養後、十分に分離されたコロニーを選択し、白金耳を使用して4〜5mLのTSAブロスを含むチューブに移しました。ブロスは、マクファーランドスケールで濁度0.5に達するか超えるまで、37°Cで一晩再度インキュベートしました。次に、接種物の濁度を、タービジメーターを使用して、マクファーランドスケールで最大0.5までの生理食塩水で調整した。調製した懸濁液には約1×10 8 が含まれていました。 10 7 の最終接種濃度を得るために1:10に希釈されたCFU / mL CFU / mL。 TSAプレートに各接種材料を均一に播種しました。次に、シート(10×10 mm 2 )1、3、および5%の濃度のナノコンポジットとコンポジット材料、およびPBATコントロールを、TSAプレートの表面に配置し、それらが十分に接着していることを確認しました。最後に、プレートをオーブンに入れ、37°Cで24時間インキュベートして、PBATサンプルの阻害を観察しました。
成長速度論法の第2段階は、定性的試験で接触阻害が明らかなナノコンポジットと複合材料のみで実施された定量的試験で構成されていました。無菌性を維持するために、1200シリーズタイプA2生物学的安全キャビネット(ThermoFisher Scientific、米国マサチューセッツ州ウォルサム)を使用してテストを実施しました。最初に、サンプルを滅菌ペトリ皿の中に置き、バイオセーフティキャビネットに持って行き、それぞれの側で15分間UV光にさらすことによってサンプルを前処理しました。次に、各菌株の24時間細菌培養物を、マクファーランドスケールで濁度0.5に調整し、続いて6つの段階希釈(1、2、3、4、5、および6)を作成しました。希釈率4、5、および6(3回)で初期カウントを実行して、時間ゼロでのカウントを決定しました。
各評価時間(2、4、6、および8時間)および各菌株に1つずつ、ウェットチャンバーを、滅菌蒸留水で湿らせた滅菌ガーゼを滅菌ペトリ皿に入れることによって準備しました。次に、上面が湿ったガーゼに触れないように、滅菌スライドを各湿ったチャンバー内に置いた。次に、3つの1×1-cm 2 ナノコンポジットと複合材料のシート、およびコントロールとしてのPBATシートを、滅菌クランプを使用してチャンバー内に配置しました。希釈液(20μL)を各正方形のシートに沈着させ、チャンバーを37°Cで2、4、6、および8時間インキュベートしました。
インキュベーション後、ウェットチャンバーを抽出し、各ポリマーシートを1mLの滅菌蒸留水を入れたファルコンチューブ内に堆積させました。チューブを2〜5分間ボルテックスしました[35]。ファルコンチューブ内の製品から3つの希釈液を作成しました。 TSAを含むペトリ皿は4つの部分に分けられました。 3つの希釈液のそれぞれを約3〜5滴(20μLに相当)、希釈していないファルコンチューブの内容物を1滴象限に配置しました。液滴がほぼ瞬時に吸収されるように、寒天プレートは完全に乾燥していなければなりませんでした。次にプレートを37℃で24時間インキュベートした後、コロニーカウンターでコロニーをカウントしました。得られたデータに使用した希釈係数を掛け、対数関数または生存率を使用してグラフにプロットしました。
結果と考察
レオメトリーは、ナノコンポジットが処理された実際の条件に近い条件下で、ナノコンポジットのレオロジー特性の動的測定値を取得するために使用されます。このために、溶融混合中の粘度の変化を制御するための測定が行われました。これらの測定結果は、追加ファイル1:図S5に示されています。モータートルクの増加は、ポリマーの溶融粘度に関連しており[21、36]、4分間の混合後に値は一定になり始めます。これは、この作業で確立された7分の混合時間が完全な混合を達成するのに十分であることを確認します。
PBATおよびNCs-PBAT / Cu 1%マトリックスのトルク値は約19.86 Nmでした。曲線(追加ファイル1:図S5)は、1%濃度のCu-NPがマトリックスの機械的特性にほとんど影響を与えなかったことを示していますが、NC-PBAT / Cu3では18.4および17.4Nmの低い平衡トルク値が得られました。 %およびNCs-PBAT / Cu 5%、それぞれ。これらの結果は、NCs-PBAT / Cuの加工性がPBATマトリックスに関して改善されたことを明確に示しています[37]。 NC-PBAT / Cu | Cu 2 の混合物でも同様の結果が得られました。 O、ここで、平衡トルク値は、負荷率が3%に増加すると減少しましたが、5%の負荷では、Cu | Cu 2 の1%の負荷の値に非常に近い値が得られました。 O-NP。平衡トルク値は、1、3、および5%でそれぞれ19.39、19.07、および19.37Nmでした。 MCの場合-PBAT / CuSO 4 混合物では、平衡トルク値はCuSO 4 の負荷として増加しました 増加しました。つまり、1%の場合は18.71 N m、3%の場合は19.16 N m、5%の負荷の場合は19.79 Nmです。この動作は、CuSO 4 のサイズに起因する可能性があります 結晶。同時に、追加ファイル1:図S5は、すべてのナノコンポジットとコンポジット材料の平衡トルクが混合時間の増加に伴って安定していることを示しています。これは、おそらくナノ粒子がポリマー鎖とほとんどの場合、混合プロセスで自己潤滑を実行します[37]。
形態学的および構造的特性
まず、化学還元によって得られたナノ粒子を分析しました。 Cu | Cu 2 の合成結果 O-NPを図1bに示します。 TEM顕微鏡写真は、球状粒子と多面体粒子の混合物を示しています。球状ナノ粒子の平均直径は26nmでしたが(図1c)、多面体ナノ粒子の直径は80〜160nmの範囲でした。これらのナノ粒子の組成は、選択領域電子回折(SAED)(図1c)によって決定され、金属CuおよびCu 2 に対応する相が見つかりました。 O.この発見は、図1aに示す回折図によって裏付けられました。 2θ=36.3°、42.17°、43.42°、50.63°、61.47°、および74.37°に6つの回折ピークがはっきりと観察されました。ナノ粒子はCuSO 4 を化学的に還元することによって合成されたためです CuOに対して、X線粉末回折パターンのX’PertHighScoreデータベースのCuのデータによって回折ピークが検証されました。 2θ=43.2°、50.63°、および74.37°のピークは、金属Cu回折面(111)、(200)、および(220)に属することが観察されました。他の3つのピークは、合成されたナノ粒子が複数の物質を含んでいたことを示しているため、回折パターンは両方の組み合わせです。 Wijesundera [38]はCu 2 の薄膜を分析しました OはXRDを使用し、2θ=36.3°、42.17°、および61.47°で回折された平面がミラー指数(111)、(200)、および(220)に対応することを示しました。これらのインデックスは、Cu 2 の構造と一致する、反フルオライト構造の中央領域の一部に対応する面心立方構造(FCC)に属しています。 O、SAED分析の結果による。
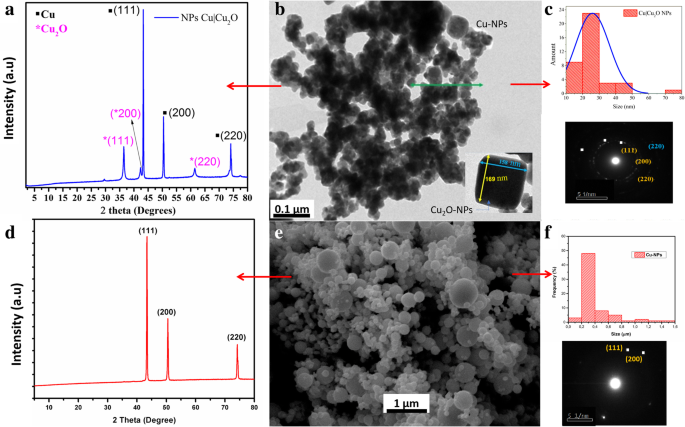
a 合成されたCuおよびCuO2ナノ粒子のXRD。 b 、 c 合成されたナノ粒子のTEM画像、サイズ分布、および回折パターン。 d Cuナノ粒子のXRD。 e 、 f Cuナノ粒子のTEM画像、サイズ分布、および回折パターン
王ら。 [39]は、化学還元によるCu-NPの合成中に、粒子のサイズが100〜150nmの範囲であることを発見しました。彼らはC 6 を使用しました H 8 O 6 還元剤として、そして界面活性剤としてポリ(ビニルピロリドン)(PVP)として。面はCu 2 の面と一致しませんでした Oなぜなら、PVPは成長中の種子を安定させ、その酸化を回避するのに役立ったからです。ただし、調査の目的はCu 2 を合成することでした。 O NP、PVPなどの安定剤を使用せずに化学還元によって達成できます。
ナノコンポジットの調製に使用されたCu-NPは球形で、直径は100〜200 nmの範囲でした(図1e、f)。図1dに示すCu-NPのXRDパターンでは、43.60°、50.72°、73.95°で明確に観察された3つのピークは、それぞれ結晶面(111)、(200)、(220)に対応しています。 Fm3m空間群(JCPDS No.85-1326)[55]の立方晶構造は、SAED分析で見つかった構造と一致しています(図1d)。
供給業者によると、我々の研究で使用された金属粒子は、機械的粉砕システムによって得られた。この方法の欠点は、粒子のわずかな割合(〜10%)が500nmより大きいことです。しかし、これは私たちの調査の目的に悪影響を及ぼしませんでした。以下に、この分散がPBATマトリックスの熱機械的特性にどのように影響したかを示します。重要なことに、機械的粉砕法は、化学還元法として知られている湿式合成法の場合のように、前駆体または安定剤を使用しません。したがって、粉砕によって得られたCu-NPの表面は、安定剤または反応副生成物のいずれかからの分子の吸着によって不動態化されません。したがって、これらのCu-NPは、ポリマーの機械的特性を実質的に改善することはありませんが、劣化させることもありません。ただし、Cu 2+ の移行のため、抗菌特性を改善する必要があります。 不動態化されていない表面で促進されます。
図2は、NCs-PBAT / Cu(図2a)、NCs-PBAT / Cu | Cu 2 のXRDスペクトルを示しています。 O(図2b)、およびMC-PBAT / CuSO 4 (図2c)。図2cは、3つの濃度(1、2、および3% w )で作成されました。 / w )。これらの回折図をPBATポリマーマトリックスの回折図と比較して、ポリマー構造に対する負荷の影響を示しました。 PBAT回折図は、平面(011)、(010)、(101)、(100)、および25°に対応する2θ=16.1°、17.3°、20.2°、23.1°、および25°に5つの回折ピークを持つ回折パターンを示しました。 (111)、それぞれ。この分析により、ポリマーマトリックスに結晶化度が存在することが明らかになりました。 ArrudaらによるPBATの特性評価。 [40] XRDを使用すると、同じ平面に対応する、この調査で見つかったものと同じ角度で同じ5つの回折ピークも見つかりました。
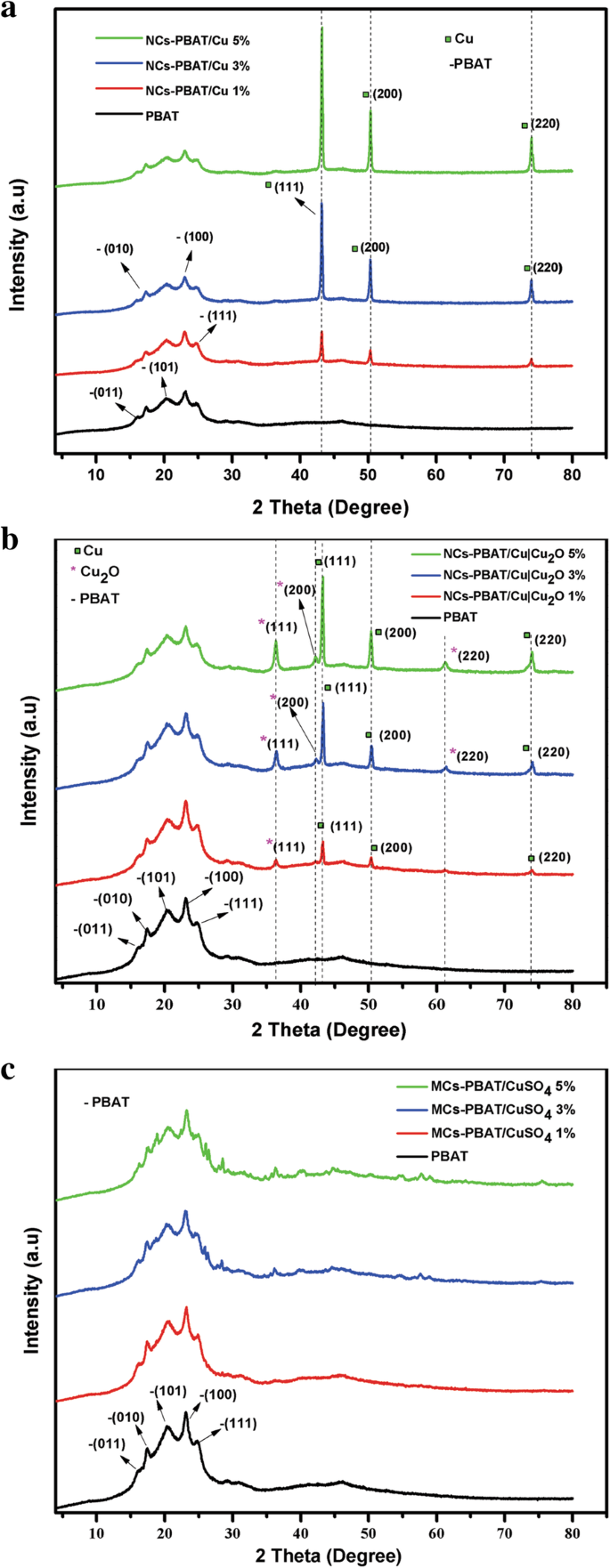
PBAT、NCs-PBAT / Cu、NCs-PBAT / Cu | Cu 2 の回折図 O、およびMC-PBAT / CuSO 4
Cu-NP負荷のあるナノコンポジットの回折図を図2aに示します。 43°、50°、および74°での2θ信号は、Fm3m空間群(JCPDS No.85-1326)を持つCuのFCC構造の平面(111)、(200)、および(220)に特徴的です。 41]。 CuOまたはCu 2 に対応する相はありません NCs-PBAT / Cuの回折図でOが観察されたため、ナノコンポジットの合成中にナノ粒子が酸化されなかったと結論付けました。さらに、回折図は、ナノ粒子がPBATの構造に影響を与えたり変更したりせず、ピークの強度がCu-NPの負荷率に正比例することを示しています。 NCの回折図-PBAT / Cu | Cu 2 Oには、2θ=36.4°、43°、42.4°、50°、61.5°、および74°に6つの特徴的なピークがあります(図2b)。文献とナノ粒子の分析によると、金属Cuに対応するのは3つだけであり、36.4°、42.4°、および61.5°のピークはCu 2 に属します。 O、図1a [35]に示されているこのタイプのナノ粒子のスペクトルによると。
Cu | Cu 2 に対応する回折ピーク O-NPの強化は、マトリックス内の濃度が高くなるにつれて強くなりましたが、ポリマーの結晶ゾーンに属するピークは、荷重を加えると強度がわずかに低下しました。 Chivrac etal。 [42]は、PBATで大量のナノクレイを使用した研究で同様の結果を報告しました。彼らは、負荷とポリマーの界面に有意な超結晶性がなく、したがって、ポリマーの結晶構造に変化がないことを示唆しました。ただし、マトリックス内の負荷の濃度の増加に伴うPBATの回折ピークの強度の減少は、PBATの結晶化度の低下を示しています。したがって、負荷はPBATの結晶成長を妨げます。これは、Cu | Cu 2 の増加に伴うPBATに属する回折ピークのわずかな減少を説明することができます。 O-NP。
図2cは、MC-PBAT / CuSO 4 のXRDスペクトルを示しています。 CuSO 4 の3つの濃度の場合 1、3、および5%の。 1%CuSO 4 の追加 負荷はポリマーに変化を生じませんでした。 3%および5%CuSO 4 負荷曲線は、CuおよびCu 2 に属する2θ=36.4°、40.25°、43.94°、57.9°、および75.7°のピークの強度の最小の増加のみを示しています。 Oが存在し、Cu 2 の一部であることを示します SO 4 混合プロセス中に還元および酸化された。 PBATの結晶ゾーンについては、CuSO 4 の濃度の増加 NCs-PBAT / CuおよびNCs-PBAT / Cu | Cu 2 で発生したように、強化によりPBATの回折ピークの強度が減少しました。 O.したがって、CuSO 4 の組み込み おそらくCuSO 4 が原因で、ポリマーマトリックスに結晶化能力が低下しました。 微結晶の成長を妨げます。 CuSO 4 のXRDスペクトルに関する追加情報がないため 複合材料で報告されているので、生分解性ポリマーでの挙動を調査する必要があります。マトリックスの結晶化度は次のように計算されました:
$$ {X} _ {\ mathrm {c}} =\ frac {I _ {\ mathrm {c}}} {I _ {\ mathrm {c}} + {I} _ {\ mathrm {a}}} $$ (1)ここで私 c は結晶相のピークの面積であり、 I c + 私 a は、回折図の下の総面積です。各材料の結晶化度の値を表1に示します。これらの結果は、Cu-NPとCu | Cu 2 の濃度が高くなるにつれて結晶化度のパーセンテージが増加することを示しています。 O-NPはPBATマトリックスで増加します。これは、それぞれの回折図のピークの強度が増加することで明らかです。
<図> 図>一方、回折図は、ナノ粒子がPBATの構造に影響を与えたり変更したりせず、ピークの強度がCu-NPとCu | Cu 2 の負荷率に正比例することを示しています。サブ> O-NP。さらに、CuSO 4 の追加 前駆体塩は、純粋な状態のポリマーの結晶化度と比較して、ポリマーの結晶化度を低下させた。この状態は、ナノコンポジットに負荷濃度を追加すると、結晶化度の相対的な割合が増加したが、PBATの結晶化度が低下したために発生しました。その結果、一般に、結晶化度の合計の割合がわずかに増加したと報告されました。 MC-PBAT / CuSO 4 負荷は、XRDスペクトルに結晶ピークを示しませんでした。したがって、それらは結晶化度の増加に寄与しませんでしたが、ポリマー鎖の結晶化度の減少を引き起こしました。これは、複合材料の結晶化度の合計パーセンテージの減少を説明しています。いくつかの研究は、金属ナノ粒子がポリマー鎖の配向において核形成の中心として機能し、それがポリマーの結晶化度を増加させることを示しています[43]。
The FTIR (Additional file 1:Figure S6) spectra show that the characteristic peaks at different load concentrations are at the same frequency but have different intensities. The spectra show that as the concentration of nanoparticles in the polymer matrix increased, the intensity of the peaks corresponding to NCs-PBAT/Cu and NCs-PBAT/Cu|Cu2 O increased with respect to the PBAT. Therefore, there was no effective interaction between the chains of the PBAT and the nanoparticles. Had there been interaction, some of the signals in the FTIR spectrum would have been displaced as a result of the interaction of the functional groups of the polymer with the surface of the PBAT [40].
Mechanical Properties (Tensile Test)
To give multifunctionality to biopolymers, nanomaterials that provide special properties to a nanocomposite are usually incorporated. Their inclusion will change the mechanical properties of the material and the intensity of the changes is directly related to the union of the nanostructure with the polymer network [44]. We conducted tensile tests on the nanocomposites and the composite material. The tensile strength and maximum deformation values are summarized in Table 2.
<図> 図>Figure 3 shows the average curves of the tensile tests on the nanocomposites and composite material. As the permanent deformation of the material began, the effect of the concentration of the nanoparticles in the polymer could be distinguished. Figure 3a shows the results for NCs-PBAT/Cu. The results show that the inclusion of nanostructures did not considerably affect the elastic range but there were noticeable changes in the yield strength. As the concentration of the Cu-NPs increased, maximum resistance increased and maximum elongation decreased. These changes clearly indicate that the nanostructures harden the PBAT. At 3% concentration of Cu-NPs, the tensile strength slightly increased but the elongation percentage in the fracture decreased between 30 and 35%.
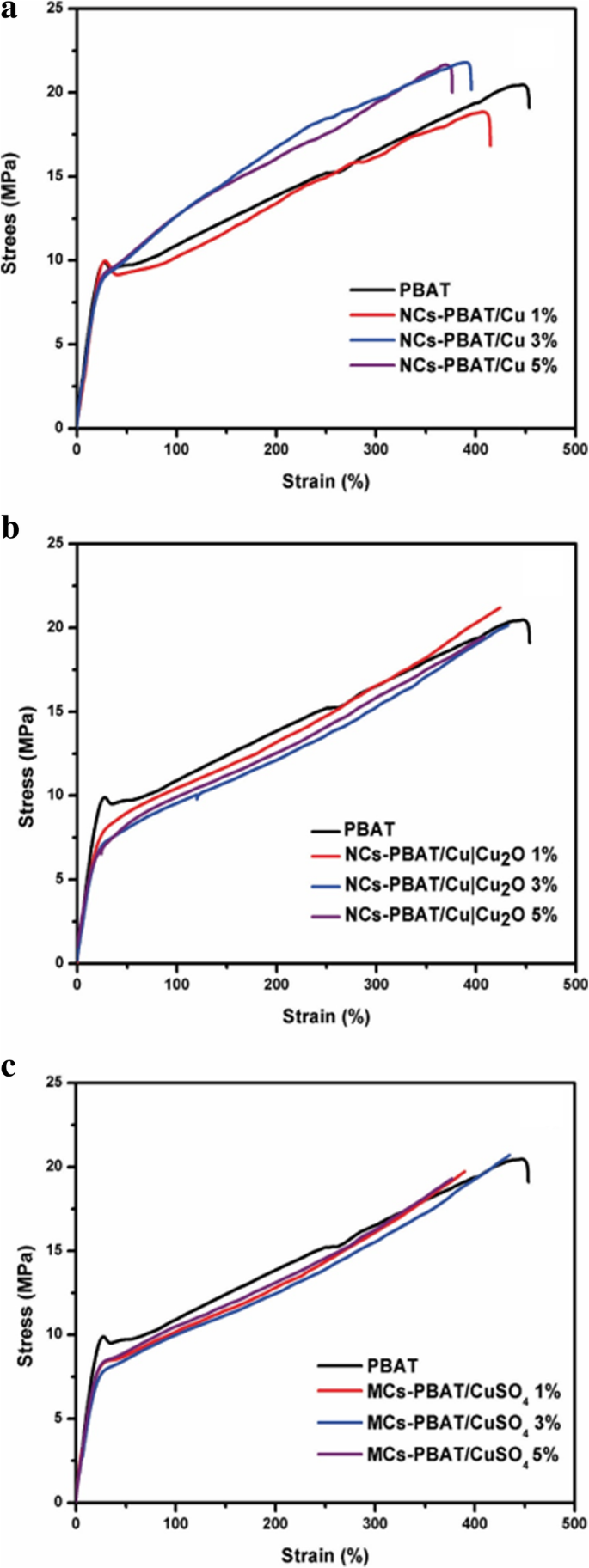
Stress and strain of PBAT, NCs-PBAT/Cu, NCs-PBAT/Cu|Cu2 O, and MCs-PBAT/CuSO4
Figure 3b shows the results of the tensile tests on the NCs-PBAT/Cu|Cu2 O. The 1% load nanocomposite clearly showed an increase in tensile strength and elongation with respect to the PBAT. There was no appreciable effect on the elastic range, but it did appear to be above the yield stress. In addition, the curve for the 3% load NCs-PBAT/Cu|Cu2 O shows there was no significant difference with respect to the PBAT. The same behavior is seen with curve for the 5% load NCs-PBAT/Cu|Cu2 O. The curves for MCs-PBAT/CuSO4 (Fig. 3c) show that the yield stress decreased for the three concentrations of CuSO4 with respect to the PBAT.
From the results, we can conclude that the reinforcements did not significantly change the mechanical properties of the PBAT. Venkatesan and Rajeswari [45] showed a significant increase in mechanical properties by incorporating ZnO nanoparticles in a PBAT matrix with respect to that of the PBAT. Similar results with some improvements were obtained by Chen and Yang [46]. They elaborated a PBAT nanocomposite with montmorillonite nanoparticles using melt blending.
Our investigation found that the NCs-PBAT/Cu|Cu2O 3 and 5% and MCs-PBAT/CuSO4 1 and 5% had slightly decreased tensile strength, that is, there were no significant variations in the mechanical properties. However, the NCs-PBAT/Cu|Cu2 O 1% and MCs-PBAT/CuSO4 3% had slightly increased tensile strength. Therefore, no reinforcement at any concentration in the matrix caused remarkable variations in the mechanical properties of the PBAT. In addition, as the concentration of Cu-NPs increased, their mechanical properties increased the resistance of the PBAT but elongation could not be maintained. The results of the tensile tests showed that the commercial Cu nanoparticles improved the tensile strength of the PBAT slightly more than did the Cu|Cu2 O nanoparticles and the CuSO4 粒子。 The difference between the tensile properties found in our investigation and those in the literature could be attributed to load dispersion because the agglomerated particles act as stress concentrators [47]. Finally, the variations in the test values were explained by the preparation conditions of the test samples, the degree of crystallinity of the PBAT, the molecular mass, the degree of interaction at the polymer-reinforcement interface, and the load dispersion because the agglomerates in the matrix could act as stress concentrators.
Thermal Properties
One of the disadvantages of the PBAT is its low thermal stability because the fusion process can degrade its polymer chains [48]. Therefore, the effect of nanometric and micrometric loads on the decomposition of this biopolymer must be investigated. TGA of NCs-PBAT/Cu, NCs-PBAT/Cu|Cu2 O, and MCs-PBAT/CuSO4 was carried out to observe the changes in the thermal stability of the PBAT caused by the presence of Cu nanoparticles in the matrix. The TGA results are shown in Fig. 4, and the initial (T di ) and final (T df ) decomposition temperatures of the analyzed samples are summarized in Table 3. The thermograms show that the polymer without any load had a weight loss of 1% at 420.77 °C, while the nanocomposites NCs-PBAT/Cu 1, 3, and 5% presented a weight loss of around 3% (Fig. 4a). This suggests that the presence of Cu-NPs at concentrations of 3 and 5% slightly increases the thermal stability of the nanocomposites compared to that of the unloaded polymer. After the final thermal decomposition, the degradation percentages, at around 420–427 °C, of the PBAT matrix and nanocomposites NCs-PBAT/Cu 1, 3, and 5% were 98.9, 97.5, 95.4, and 96.8%, respectively. The residues were higher for Cu-NPs-incorporated nanocomposite samples. Similar results have been reported for PBAT nanocomposites with different loads of Ag-NPs [49].
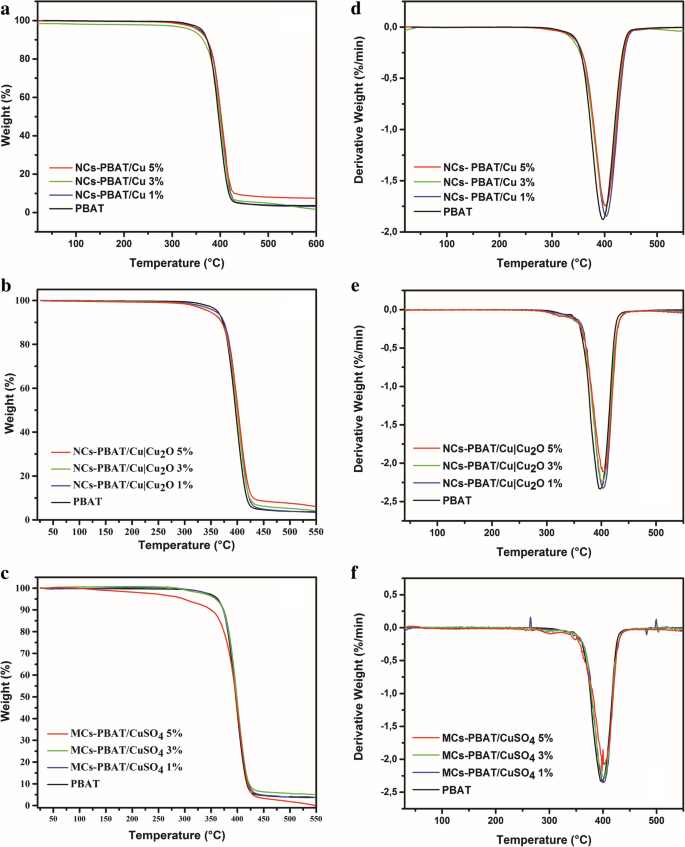
TGA of a PBAT and NCs-PBAT/Cu, b NCs-PBAT/Cu|Cu2 O, and c MCs-PBAT/CuSO4 , DTG of d PBAT and NCs-PBAT/Cu, e NCs-PBAT/Cu|Cu2 O, f MCs-PBAT/CuSO4
Although no significant change is seen among the curves in Fig. 4b for the NCs-PBAT/Cu|Cu2 O, the results show that as the Cu|Cu2 O-NPs increased in the polymer structure, T di increased and T df decreased with respect to the initial and final degradation temperatures of PBAT; in addition, the total mass loss decreased. By calculating the derivative of the mass with respect to the temperature, we obtained the curves in Fig. 4d–f for the indicated peaks of the nanocomposite with Cu|Cu2 O-NPs and found that T df , at which the maximum decomposition occurs, was between 402 and 403 °C (Table 3).
The CuSO4 loads incorporated into the polymer matrix, i.e., MCs-PBAT/CuSO4 , yielded the same behavior as that of the NCs-PBAT/Cu|Cu2 O, with an increase in T di and a decrease in T df with respect to the PBAT polymer. T di values of the NCs-PBAT/Cu|Cu2 O and the MCs-PBAT/CuSO4 were greater than that of the NCs-PBAT/Cu, but the T df and degradation percentage values were less than those of the nanocomposites with Cu-NPs loads.
This enhancement of the thermal stability of the PBAT is attributed to the barrier effect of the loads. The loads were also supposed to have a shielding effect on the matrix to slow the rate of mass loss of the decomposition product [50]. The data obtained by our analysis were compared with published results to verify that the indicated behavior is usual for this type of polymer. Sinha Ray et al. [51] found by thermal analysis of PBAT reinforced with nanoclays that the degradation temperatures of the nanocomposites were greater than or at least equal to that of the PBAT. In general, the reinforcements improve the thermal stability of the polymer matrix because they act as a heat barrier, which improves the total thermal stability of the system. However, the studies of Sinha Ray et al. and this investigation showed that the thermal stability of the nanocomposite and PBAT compounds only slightly improved. To explain the relatively low improvement in the thermal stability of some nanocomposites, Sinha Ray et al. assumed that in the early stages of thermal decomposition, the reinforcements displace the decomposition to higher temperatures, but in a second stage, the clay layers accumulate heat and then act as a source of heat. This heat source, along with the heat flow supplied by the external heat source, promotes the acceleration of decomposition. This could explain the behavior of the reinforcements in the NCs-PBAT/Cu|Cu2 O and MCs-PBAT/CuSO4 。 Thus, we conclude that the thermal properties of the nanocomposites and the composite material slightly improve but not significantly. On the other hand, the results of DSC (Additional file 1:Figure S7 and Table S1) indicated that the addition of reinforcements to the matrix slightly hindered the kinetics and degree of crystallization of the PBAT. The addition of clays increased the crystallization temperature from 1 to 10 °C and the melting temperature from 1 to 5 °C. These phenomena were probably due to an increase in the viscosity of the polymer with the addition of clays, which reduced the mobility of the macromolecular chains against the growth of crystals.
Comparative Evaluation of the Antimicrobial Activity of NCs-PBAT/Cu, NCs-PBAT/Cu|Cu2 O, and MCs-PBAT/CuSO4
Qualitative Test
After the experimental procedure was performed, we wanted to observe whether bacterial colonies were inhibited by each PBAT sample, i.e., NCs-PBAT/Cu 1, 3, and 5%; NCs-PBAT/Cu|Cu2 O 1, 3, and 5%; and MCs-PBAT/CuSO4 1, 3, and 5%. We decided to use the 3% concentrations because the 1% concentrations did not produce enough bacterial inhibition and the 5% concentration produced behavior similar to that of the 3% concentration, the minimum percentage with activity that avoided toxicity in the polymer.
Quantitative Test
The study was carried out at different contact times using four bacterial strains and the PBAT samples NCs-PBAT/Cu 3%, NCs-PBAT/Cu|Cu2 O 3%, and MCs-PBAT/CuSO4 3%. The times and colony-forming unit counts (CFU/mL) are presented in Table 4, and the bacterial activity and colony count for each Petri dish are shown in Fig. 5. In addition, a graphical analysis is shown in Fig. 6, where images of bacterial growth are also presented. The statistical analysis of the data is summarized in Table 5.
<図> 図>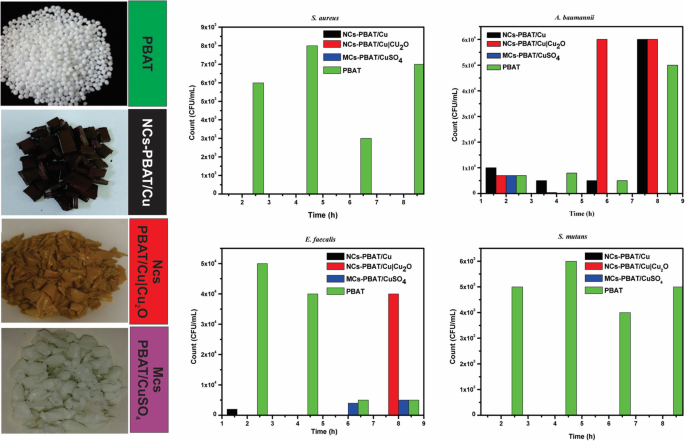
Bacterial activity and colonization count PBAT, NCs-PBAT/Cu-3%, NCs-PBAT/Cu|Cu2 O 3%, and MCs-PBAT/CuSO4 3% for each strain of bacteria. 黄色ブドウ球菌 , Acinetobacter baumanni , Enterococcus faecalis , Streptococcus mutans
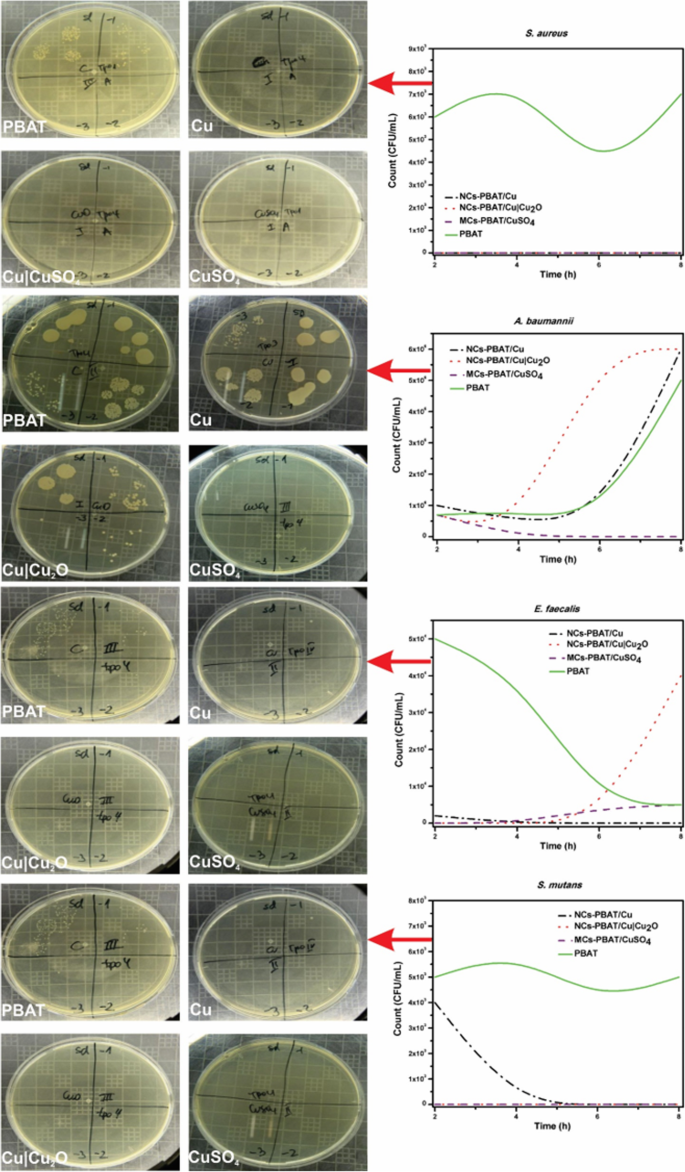
Graphical analysis of colony count (CFU/mL) vs time (h) of PBAT, NCs-PBAT/Cu-3% NCs-PBAT/Cu|Cu2 O 3%, and MCs-PBAT/CuSO4 3% for each strain of bacteria. Enterococcus faecalis , Acinetobacter baumanni , Streptococcus mutans , Staphylococcus au reus
The study of A. baumannii found that the colonies grew in all periods (2, 4, 6, and 8 h) in the samples containing Cu-NPs, Cu|Cu2 O-NPs, and PBAT. High bactericidal activity occurred with the sample containing CuSO4 during exposure times of 4, 6, and 8 h, decreasing from 7 × 10 5 to 0 CFU/mL. The sample containing Cu-NPs showed a significant increase in the growth of bacterial colonies from 1 × 10 5 to 6 × 10 6 CFU/mL, with an average of 2 × 10 6 CFU/mL. The bacterial colonies in the sample containing Cu|Cu2 O-NPs grew from 7 × 10 5 in time I to 6 × 10 6 in time IV, with an average growth of 3.19 × 10 6 CFU/mL. Bacterial growth in the PBAT reached an average of 1.75 × 10 6 CFU/mL.
The study of E. faecalis found good bactericidal activity by the samples containing Cu-NPs, Cu|Cu2 O-NPs, and CuSO4 , with average colony growth of 5 × 10 2 , 1 × 10 4 , and 2.2 × 10 3 CFU/mL, respectively, while the PBAT did not show bactericidal activity and the colonies grew at all times. Colony growth in the sample containing Cu-NPs was 2 × 10 3 CFU/mL at 2 h then dropped to zero at 4, 6, and 8 h, whereas the samples containing Cu|Cu2 O-NPs had 0 CFU/mL at times I, II, and III, but 4 × 10 4 CFU/mL at time IV. Samples containing CuSO4 prevented the growth of bacteria in times I and II with growth activity of 0 CFU/mL, but colonies grew to 4 × 10 3 and 5 × 10 3 CFU/mL for times III and IV, respectively. PBAT did not show bactericidal activity against E. faecalis 。
The study of S. mutans found no colony growth in the samples containing Cu|Cu2 O-NPs and CuSO4 。 The sample containing Cu-NPs showed very good bactericidal activity except at time I, at which colony growth was 4 × 10 3 CFU/mL, making the average growth for the four times 8 × 10 2 CFU/mL. PBAT without reinforcement showed no bactericidal activity against S. mutans 。 The samples containing Cu-NPs, Cu|Cu2 O-NPs, and CuSO4 in contact with S.アウレウス showed an excellent bactericidal response. They completely inhibited the growth of colonies, while PBAT did not show any bactericidal activity against S.アウレウス , which grew an average of 6 × 10 3 CFU/mL.
In general, the antibacterial effectiveness of polymer-and-metal nanocomposites improves with a high surface/volume ratio, which increases the number of ions released from the nanoparticles into the polymer. The mechanism of the corrosion of Cu in aqueous solutions and the resulting Cu species vary with pH. In general, the species Cu2 O and CuO are formed and can be dissolved in Cu ions. Elemental metal particles require the presence of water and oxygen molecules to release a small amount of ions. Therefore, retention of water and oxygen within the polymer is crucial for the release of Cu ions. Some properties of polymer-and-metal nanocomposites such as the crystallinity and polarity of the matrix, which constitute a barrier for the diffusion of water molecules and ions during their propagation, can affect the rate of release. Shankar and Rhim [49] prepared films composed of PBAT and Ag nanoparticles (PBAT/Ag-NPs) that showed strong antibacterial activity against E.コリ and Listeria monocytogenes compared with that of PBAT films without Ag-NPs. Similar results were obtained by Venkatesan and Rajeswari [45] when they evaluated the antimicrobial activity of ZnO-NPs incorporated in a PBAT matrix. The PBAT compound, which was used as a control matrix, showed no antimicrobial activity compared to the PBAT/ZnO-NPs nanocomposite films. The results showed that the films had high bactericidal activity against the pathogens tested (E. coli および S。アウレウス ), with increased inhibition of bacterial growth as the ZnO load concentration increased from 1 to 10% by weight. This ability of Cu, Zn, and Ag nanoparticles to inhibit bacterial growth is mainly due to the irreparable damage to the membrane of the bacterial cells caused by the interaction between the surface of the bacteria and these oxides and metals [52, 53]. Compared with the works discussed above, our investigation found significant antimicrobial activity against inpatient and oral-resistant strains.
To complement this investigation, we performed water absorption tests using three different media and following point 7.4, “Long-Term Immersion”, in ASTM D570-98. The results of these tests are reported in the supplementary material, Additional file 1:Table S2–S4 and Figure S8, with their respective analysis. Analysis showed that sulfate-based composite materials absorb large amounts of water, even in acidic and basic environments. This phenomenon greatly affects the mechanical properties of these materials; however, resistant bacteria, such as A. baumannii , require an immediate Cu + distribution to control them. This explains the antimicrobial power of CuSO4 within the PBAT matrix.
Conclusions
Using XRD and TEM, we determined that the synthesis of nanocomposites and material composites based on PBAT using chemical reduction and a mixture of metal Cu nanoparticles with CuO2 , where Cu had a spherical morphology and Cu2 O had a polyhedral morphology. The structural characterization of the NCs and MCs by FTIR and XRD showed that the Cu-NPs, Cu|Cu2 O-NPs, and CuSO4 reinforcements did not modify the structure of the PBAT. However, they did slightly alter the percentage of its crystallinity, which increased with NPs and decreased with CuSO4 。 On the other hand, the mechanical properties of the PBAT for both the NCs and MCs did not vary significantly with the addition of reinforcements, meaning that the PBAT maintained its mechanical properties. From the thermal tests, we concluded that reinforcing the PBAT did not fundamentally improve its thermal properties, it only increased its thermal stability a few degrees Celsius, which is not significant. Antimicrobial analyses showed that the Cu|Cu2 O-NPs within the PBAT generated antibacterial activity against E. faecalis および S。 mutans and excellent bactericidal properties against S.アウレウス 。 CuSO4 had a good bactericidal response against A. baumannii 、 E。 faecalis , and S mutans and an exceptional response against S.アウレウス 。 The PBAT without loads did not present bactericidal properties when in contact with the bacterial strains. In general, the addition of loads into the PBAT generates bactericidal activity that the polymer does not possess by itself. The addition of CuSO4 yielded the best antimicrobial response against the four strains used in this investigation. In the search for new applications for bionanocomposites, it will be essential to evaluate their antimicrobial response in food containers, medical devices, packaging, and other products; analyze their biocidal effects against other bacteria against which only NPs have antibacterial characteristics; and justify the expense associated with their synthesis.
略語
- Cu|Cu2 O-NPs:
-
Copper/cuprous oxide nanoparticles
- Cu-NPs:
-
Copper nanoparticles
- CuSO4 :
-
Copper sulfate
- DSC:
-
示差走査熱量測定
- FTIR:
-
フーリエ変換赤外分光法
- MC:
-
Composite material
- MCs-PBAT/CuSO4 :
-
Composite materials of poly(butylene adipate-co-terephthalate) with copper sulfate
- NCs:
-
Nanocomposites
- NCs-PBAT/Cu:
-
Nanocomposites of poly(butylene adipate-co-terephthalate) with copper nanoparticles
- NCs-PBAT/Cu|Cu2 O:
-
Nanocomposites of poly(butylene adipate-co-terephthalate) with copper/cuprous oxide nanoparticles
- PBAT:
-
Poly(butylene adipate-co-terephthalate)
- TEM:
-
透過型電子顕微鏡
- TGA:
-
熱重量分析
- XRD:
-
X線回折
ナノマテリアル
- コバルトをドープしたFeMn2O4スピネルナノ粒子の調製と磁気特性
- MnХFe3−XО4スピネルの構造的および磁気的特性に及ぼす接触非平衡プラズマの影響
- フェニルトリメトキシシランで修飾されたアルミナナノ粒子をベースにしたAl2O3:SiOCナノコンポジットの形成と発光特性
- フラーレン由来のナノ材料とそれらのポリマー複合材料の常磁性特性:劇的なポンピングアウト効果
- Cu / Ti3SiC2 / Cナノコンポジットの微細構造と機械的性質に及ぼすグラフェンとMWCNTの相乗効果
- 磁性ポリ(N-イソプロピルアクリルアミド)ナノコンポジット:抗菌特性に及ぼす調製方法の影響
- ポリ(N-ビニル-2-ピロリドン)燃焼におけるPdクラスターの触媒効果
- ポリマーナノコンポジットのヤング率に対するナノ粒子の凝集/凝集の影響を研究するための2段階の方法論
- <100>引張荷重下のタンタル単結晶の弾性特性の温度および圧力依存性:分子動力学研究
- 天然および合成ナノ材料の電気化学的、生物医学的、および熱的特性の比較研究
- 金属および金属酸化物ナノ粒子のグリーン合成と単細胞藻類Chlamydomonasreinhardtiiに対するそれらの効果