木材ベースの製品産業におけるナノテクノロジーの応用:レビュー
要約
木材産業は、マレーシアの経済成長の主な推進力の1つです。さまざまなリグノセルロース材料の供給源である森林には、木材産業自体または多くの異なる用途分野で使用するための非常に興味深い機能を備えた、持続可能で生分解性のナノサイズの材料を生産するために利用できる多くの未開発の可能性があります。木材ベースの製品部門は、既存の製品の性能を向上させたり、森林から新しい付加価値製品を作成したりするために、さまざまな容易に入手可能なナノ材料を利用することもできます。このレビューは、木材ベースの製品産業におけるナノテクノロジーアプリケーションの最近の発展に焦点を当てています。
はじめに
ナノテクノロジーは、1〜100nmの物質の操作として定義されています。ナノテクノロジーは、科学と技術を組み合わせた学際的な分野であり、重要な機能、物理的および化学的特性を備えた新しく改良された材料の開発を目的としています[1、2]。このスケールの材料は、高次元の同じ材料と比較して、独特の特性を持っています[3]。このため、ナノテクノロジーによるさまざまな革新的なアプリケーションを多くの分野で検討することができます。
ナノテクノロジーは、テクノロジーイネーブラーとしてだけでなく、国家の経済成長を達成するための推進力にもなり得ます。マレーシアの林産物産業は、2019年に約RM225億の輸出額に貢献しています[4]。マレーシアから輸出される木材製品には、製材、ベニヤ、合板、モールディングなどがあります。マレーシアの木材産業は、ナノテクノロジーを十分に活用して独自の利点を得る機会があります。既存の木材製品を多様化し、付加価値を付け、最終的にこの分野の経済成長を後押しするためには、特にマレーシアの木材産業にナノテクノロジーを適用する取り組みが支援され、注目されることが不可欠です。
森林が長年にわたって文明に不可欠な役割を果たしているという事実は、さまざまな製品の開発や開発のためのリグノセルロース材料の供給源になっているため、除外することはできません。実際のところ、木材製品は、木材、家具、紙、その他多くの機能性材料の形で社会で長い間利用可能でした[5、6]。林業部門、特に林産物または木材ベースの製品におけるナノテクノロジーの適用は、すべての関連するプレーヤーおよび利害関係者からの注意を必要とします。ナノテクノロジーの利用は、より強力で多機能でありながら軽量の木材ベースの製品をもたらす可能性があります[3]。パルプや紙、木材複合材、木材コーティング、木材防腐剤などの従来の林産物は、拡張または新しいまたは付加価値のある製品に変換して、より幅広いおよび/またはより高度な用途を見つけることができます[5]。
このレビューペーパーでは、木材ベースの製品産業におけるナノテクノロジーの適用は、2つの[2]経路に分けることができます。
- 1
森林からのナノマテリアルの派生
環境に対する懸念の高まりにより、持続可能で生分解性のナノサイズの材料を導入する必要性が高まっています。ナノセルロースと呼ばれるこの新しい材料は、安全で持続可能な方法で森林資源から生産することができます。森林からのリグノセルロース材料の豊富さは、セルロースをナノセルロースに変換することへの関心の高まりを生み出しました。ナノセルロースは主に分子レベルのセルロースであり、高強度と剛性、高強度対重量比、電磁応答、大きな表面積などのいくつかの重要な機能を備えています[5、7、8、9]。この材料の優れた特性は、林産物産業自体を超えた巨大なアプリケーションの可能性を提供します。木材ベースの製品分野では、パルプおよび紙と木材の複合材料の補強剤として、または木材コーティングのコーティング材料として適用できます[10、11、12]。ナノセルロースはその汎用性により、電子機器、センサー、電池、食品、医薬品、化粧品などの非林産物セクターのコンポーネントの1つとして(基板、安定剤、電極として)使用できます[5、13]。
> - 2
木質製品へのナノマテリアルの使用
ナノマテリアルは、その機能性の観点から既存の木質製品を強化するために使用できます。たとえば、ナノ亜鉛酸化物やナノチタン酸化物などの木材コーティングにナノ材料を使用すると、耐久性、耐火性、UV吸収、および吸水率の低下という点で、木材の機能を強化できます[14、15、16]。一方、木材防腐剤へのナノカプセル化の適用[17、18]は、化学物質が木材の奥深くまで浸透し、過度の浸出の問題を減らすことにより、農薬による木材の含浸を改善する可能性があります[19]。これにより、生分解剤に対する処理済み木材の耐久性が向上します。
林業および林産物におけるナノテクノロジーの応用に関する多くの総説が発表されています[20、21]。 McCrankによる記事[21]は、森林部門におけるナノテクノロジーの応用の全体的な概要を示していますが、Moon etal。 [20]ナノインデンテーションについてさらに議論します。著者の知る限り、このトピックに関する出版物は限られており、特に木材産業の選択されたセクターをカバーしています。このレビューペーパーは、選択された木材ベースのセクター、すなわちパルプと紙、木材複合材、木材コーティング、および木材耐久性におけるナノテクノロジーアプリケーションの最近の進歩に焦点を当てます。さらに、木質製品産業における新世代のセルロースであるナノセルロースのいくつかの潜在的な用途も、エネルギーやセンサーなどの分野で強調されています。
アプリケーション
紙パルプ
世界中の紙と板紙の年間生産量は4億トン以上です[22]。これは、現在のデジタル時代が地域社会での紙製品の継続的な使用を止めていないことを示しています。それにもかかわらず、需要の高まりは主にさまざまな包装製品に対するものです[23]。この上昇は、オンラインショッピングに対する消費者の嗜好の変化と、自宅で快適に買い物をするための便利さを提供し、目的の受信者に製品を安全に届ける電子商取引の促進によって促進される可能性があります。
紙は、一連の一次処理、処理、製紙、乾燥、コーティングを経たリグノセルロース材料から作られています。製紙段階では、パルプと添加剤のすべての混合が追加され、さまざまな最終製品に合わせて調整されます。ナノテクノロジーは、ナノ材料またはナノ添加剤の形で紙パルプに適用されます。たとえば、森林資源またはむしろリグノセルロース材料を利用して、ナノサイズのビルディングブロックまたはナノセルロースとして知られる、独自の機械的強度、機能性、および柔軟性を提供する補強ユニットとして機能するものを抽出できます[5]。この新世代のセルロースは、細胞壁の剥離によってナノフィブリルを取得するか、ナノスケールで結晶セルロースを抽出することによって生成されます。ナノセルロースは、主に紙の強度を高めるためにペーパーメイキングに追加されます。製紙プロセスで使用される他のナノ材料には、ナノシリカ、ナノゼオライトなどがあります[22]。製紙業界にナノ材料またはナノ添加剤を組み込むことで、紙製品の性能を向上させることができます。
紙パルプ産業で使用されている、または使用される可能性のある主な添加剤については、次のセクションで説明します。
湿式または乾式強度剤としてのナノセルロース
ナノセルロースは、紙パルプ分野で強度添加剤として広く研究されてきました[24、25]。ナノセルロースは、その豊富さ、入手可能性、および生分解性、低毒性、優れた機械的および光学的特性、高表面積、再生可能性などの興味深い特性により、大きな関心を集めています[5、7]。ナノセルロースは、実際にはナノスケールの寸法のセルロースであり、木材パルプ、非木材植物、木材および農業残渣、細菌性セルロースおよびチュニケートを含む任意のリグノセルロース材料から単離および調製することができます。セルロースは、結晶領域とアモルファス領域を含むβ-1,4結合グルコース単位を持つ線状化合物です。鉱酸を使用してセルロース鎖からアモルファスドメインを除去すると、ナノ結晶セルロースが分離されますが、高せん断の機械的作用による細胞壁の剥離により、セルロースの幅が狭くなり、ナノフィブリル化セルロースが形成されます。ナノセルロースのこれらの2つのカテゴリーは、製品強化のための紙パルプ分野で最も研究されているナノ材料です。過去10年間、さまざまな植物資源からのナノフィブリル化セルロース(NFC)およびナノ結晶セルロース(NCC)の調製に多くの研究が集中してきました。図1は、透過型電子顕微鏡(TEM)を使用したナノ結晶セルロースとナノフィブリル化セルロースの画像を示しています。
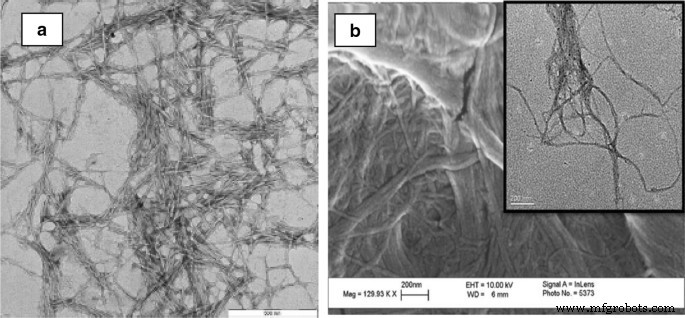
Acacia magium からのNCCのTEM画像 a ソース :ジャスマニとアドナン[26]。エルゼビアとNFCの許可を得て針葉樹パルプから複製 b ソース :趙ら[27]。エルゼビアの許可を得て複製
ナノセルロースの特性は、セルロースの調製方法と供給源によって異なります。表1は、ナノフィブリル化セルロースとナノ結晶セルロースの形態と結晶化度を示しています。
<図> 図>セルロースからナノセルロースへ
多くの研究が報告されているため、ナノフィブリル化セルロースはペーパーメイキングで最も研究されているナノ添加剤です[3、25]。ナノフィブリル化セルロースは、繊維を選択的な高せん断機械的処理にかけることによって調製されます。ナノフィブリル化セルロースを製造するための多くの方法および方法の組み合わせがあります。均質化とマイクロ流動化は、ナノフィブリル化セルロースの調製に使用される一般的な手法であり、通常10〜15回のパスを繰り返すために高圧下で繊維を小さなノズルに入れる必要があります[32、33]。このタイプの処理を単独で使用する場合は、高いエネルギー消費が必要になるため、TEMPO(2,2,6,6-テトラメチルピペリジン-1-オキシル)を介した酸化[34]、カルボキシメチル化、または酵素[35、36 ]通常、エネルギーを節約するために必要です。この方法を使用すると、流体の流れからの高速と力によって引き起こされる高いせん断速度の結果として、ナノフィブリル化セルロースが生成されます。ナノフィブリル化セルロースを製造するための別の一般的な方法は、粉砕法によるものです。研削盤は、静的および回転する石のディスクで構成されています。石によって生成されたせん断力は、細胞壁繊維を個別化されたナノフィブリルに分解します。凝集によるこの方法を使用した幅の広い分布を回避するために[37]、著者は、パルプ化および漂白プロセスの後に原料を水中に保持する必要があることを推奨しました。これは、乾燥状態にあるときに水素結合ネットワークの形成を妨げるためです[37、38]。超音波処理法もナノファイバーを調製するために研究されてきました。超音波処理には、ファイバーセル構造を破壊する可能性のある超音波からのキャビテーションによる高せん断力の生成が含まれます[39]。超音波処理技術は、十分に分散された安定したナノファイバーを生成すると言われています[3]。それに加えて、低温破砕を適用して、天然繊維をナノファイバーに変換することもできます。繊維は、液体窒素とそれに続く高せん断力を使用して凍結する必要があります。高いせん断力が氷晶に圧力を引き起こし、細胞壁を破裂させてミクロフィブリルを放出させます[40,41,42,43]。
天然セルロースから抽出されたナノ結晶セルロースは、通常、酸加水分解を使用して調製されます。 TEMPOを介した酸化[44]やイオン液体[45]などの特定の化学物質の使用を含む他の方法も、ナノセルロースの調製のために研究されています。セルラーゼ酵素の適用も報告されています[46、47]。
酸加水分解中、セルロースの重合度は急速に低下しますが、重合度のレベルオフとして知られるある時点で安定します[48]。この挙動の理由は、アモルファス領域が酸によって急速に加水分解されるためである可能性があります。加水分解が始まると、酸はその体積が大きいためにアモルファス領域を攻撃し、容易にアクセスできるグリコシド結合を加水分解することが好ましい[49]。容易にアクセスできる結合を加水分解すると、グルコース鎖の還元末端および結晶領域表面で、はるかに遅い速度でさらに加水分解が起こります[50]。酸加水分解は、酸の種類、酸濃度、温度、時間などの要因の影響を受けます[49、51、52]。酸濃度、加水分解時間、および温度条件の変化は、ナノ結晶セルロースの形態に影響を及ぼします。たとえば、加水分解時間[53]と酸濃度[29]の両方を増やすと、ナノ結晶セルロースが短くなりますが、高温ではセルロースがグルコースに完全に変換されます[50、54、55]。
製紙にナノセルロースを添加すると、紙の強度[56、57]と密度[58,59,60]が向上し、気孔率も低下する[61]という点で、より優れた性能の紙が得られます。ナノ構造のセルロースを添加すると、打たれた繊維で同様の紙の特性が追加されます[3、25]。理論的には、紙の強度は、湿式および乾式強度剤の含有[24、62]、官能化繊維の添加[63]、および叩解[64]によって高めることができます。強化により、繊維結合能力が得られます[25]。 Boufi etal。 [25]提案されたメカニズムは、繊維間の結合につながる繊維間のコネクターとして作用するナノセルロースの結果としての結合面積の増加に起因する可能性があります。それに加えて、それはまた、結合能力の増加をもたらすファイバーで作成された異なるネットワークが原因である可能性があります。ナノセルロースの長さがマイクロメートルであることを考えると、それらは隣接する繊維を接続するためのブリッジとして機能し、より強力なネットワークにつながります[25]。繊維とナノセルロースに起因する相互結合ネットワークは、紙の強度を高めます。
ナノ添加剤としてのナノセルロースは、内部結合を改善し、その結果、乾燥引張強度が増加し、通気性と不透明度が低下し、密度が高くなります[3]。ナノスケールの材料であるナノセルロースは表面積が大きいため、水素結合をより効果的に形成することができます。乾式強度の向上と保持力の向上のために、ウェットエンド添加剤として添加されます。乾燥強度添加剤に一般的に使用されるナノセルロースは、ナノフィブリル化セルロースの形をしています。
NFCをパルプファニッシュに追加するには、[24、62、65]を使用して直接追加するか、保持補助剤を使用せずに[58]、または他のフィラーや長繊維と混合して[66、67]保持補助剤を使用するため、さまざまな戦略があります。 。 NFC添加後の引張強度の増加は、添加量に関係します。打たれた歯髄とカチオン性澱粉からなる歯髄に3%のNFCを添加すると、5%の引張強度の増加が観察されることがわかった[24]。歯髄の打撃が少ないほど効果が顕著であることに注目するのは興味深いことです。たとえば、熱機械パルプに6%のNFCを添加すると、引張強度が100%以上向上します。一方、NFCは、よく叩かれた化学パルプに添加した場合の影響は小さかった[65]。したがって、NFCを充填紙、機械パルプ、再生パルプに添加すると、引張りの向上が見込まれます。 Hii etal。 [65]は、NFCがフィラーとファイバーに吸着され、フィラーをファイバーネットワークでブリッジするという観察結果を報告しました。ペーパーメイキングでNFCを使用することの唯一の大きな欠点は、排水が遅くなることです。排水は、製紙の効率に直接関係するため、製紙において非常に重要な役割を果たします。排水時間が遅いほど、紙の生成も遅くなります。したがって、特定の投与量で保持剤を使用して、繊維の表面へのナノファイバーの吸着を改善し、脱水を改善することが非常に重要です[24、68]。
NFCは、でんぷんなどの乾燥強度添加剤と、叩く作用で生成される微粉の中間の特性を持っており、どちらも結合面積を増加させます[3]。これは、繊維表面に柔らかく薄い層を作成することで実現され、乾燥中の繊維の結合を促進し、繊維間の空隙や細孔を埋めて結合面積を増加させます[3]。
バリア特性のコーティング材料としてのナノセルロース
ナノセルロースはバリア性に優れているため、包装紙のコーティング材としても使用できます。コーティング要素としてナノセルロースを使用する利点は、紙が作られた後に追加されるため、脱水の問題がもはや問題にならないことです。スプレー、バーコーティング、サイズプレス、ロールコーティングなど、ナノセルロースを塗布するために使用できるさまざまなアプローチがあります。ナノセルロース、特にナノフィブリル化セルロースの適用は、酸素バリアと耐油性を高めることが報告されています[69]。一例では、通気性の低下が69,000から4.8、660から0.2 nm Pa -1 に観察されました。 ロッドコーターを使用した、無漂白紙と耐油紙のそれぞれ[70]。 SyverudとStenius [71]は、針葉樹パルプに0〜8%のさまざまな量のNFCを適用し、バリア特性が6.5〜360 nm Pa -1 に著しく増加することを発見しました。 s -1 。これは、ナノフィブリルの増加によって引き起こされる多孔性の低下によるものです。 NFCとシェラックの適用もHultらによって試みられました。 [72]紙や板紙では、通気性、酸素透過率、水蒸気透過度が低下し、バリア包装の可能性があります。
それだけでなく、ナノセルロース、特にナノフィブリル化セルロースは、自立型の薄膜またはナノペーパーに変換することができます。このナノペーパーは、透明で柔軟性があるため、電子アプリケーションの基板として使用できます[73]。
資産改善のための保持剤としてのナノマテリアル
紙への機能性化学物質の保持を改善するために、保持剤がペーパーメイキングに追加されます。ナノゼオライト[74]やナノ二酸化チタンの使用など、いくつかのナノ材料は紙製品でテストされています。 Nanozeoliteは、製紙業界で水分を吸収するための乾燥剤として使用され、特殊紙に使用される場合はガス放出を除去する機能もあります。ボイドとポアからなるナノゼオライトの高表面積は、そのようなプロセス中に役立ちます。紙に添加されたナノチタンオキシドは、制御されたサンプルと比較して、より優れた動的弾性率を備えた紙を形成することができます[75]。
プロパティ強化のためのナノフィラー効果
製紙業界でのフィラーの使用は、フィラーがパルプ自体よりも通常安価であるため、主にコスト削減によるものです。強度添加剤として研究されていることに加えて、ナノセルロースはフィラーとしても使用できます。ナノフィブリル化セルロースの添加は、木材パルプの量を減らし、フィラーの量を増やすことができるため、製造コストを削減できます[76]。さらに、製造された紙は、低気孔率および高不透明度などの強化された特性を有する。フィラーとして2〜10%のナノフィブリル化セルロースを添加すると、強度が50〜90%増加することも報告されています(Future Markets Inc.2012)。ナノクレイは、ペーパーメイキングの添加剤として使用して、ガスの透過性を低下させ、紙の貯蔵寿命を延ばすことができます。これは、ガスや水のバリアが食べ物や飲み物の腐敗を防ぐのに重要な役割を果たす包装業界では不可欠です。
ナノ炭酸カルシウムは、光散乱を改善するためのフィラーとして使用されます。ナノ構造粒子を使用して修飾された沈殿炭酸カルシウムは、光散乱にプラスの影響を及ぼしました[77]。沈殿した炭酸カルシウムは、ケイ酸塩と硫化亜鉛のナノ粒子でコーティングされていました。ワイルドら。 [78]は、ナノ粒子コーティングが実験室、パイロット、および工場の試験で使用された同様の研究を報告しました。この研究では、ナノ粒子コーティングが優れた印刷品質、耐水性、および寸法安定性をもたらすことがわかりました。紙に添加された酸化ナノ亜鉛は、紙に抗菌性を与えます。同時に、ナノ亜鉛酸化物を添加することにより、紙の明るさや白色度、印刷適性などの光学特性も向上しました。ナノチタンオキシドは、コート紙でベータシクロデキストリンと組み合わせて研究されています[79]。ナノ材料混合物は、ナノチタン酸化物のみでコーティングされた紙と比較して、キシレンに対してより優れた分解効果を有することが見出されました。
プロパティ改善のためのサイジングエージェントとしてのナノマテリアル
紙は印刷や筆記の目的に適しているように、水/液体の浸透の抵抗を改善するために、サイジング剤が製紙に追加されます。ナノシリカを使用すると、光学特性を改善し、プリントスルーを最大30%削減できます。ナノシリカでコーティングされた紙は、コーティングされていない紙よりも優れた光学密度、寸法安定性、印刷品質を生み出すことがわかりました[80,81,82]。
ウッドコンポジット
木材は、多くの用途で使用できる生分解性で再生可能な材料であるため、人類への自然の贈り物です。しかし、木材自体には、シロアリの攻撃などのために、デリケア、柔軟性がなく、耐久性がないなどのいくつかの弱点があります。木材複合材の製造に木部繊維を利用することには、かさ密度が低く、熱安定性が低く、水分を吸収する傾向が高く、生物学的分解を受けやすいため、独自の欠点があります。ナノテクノロジーは多くの科学で利用されており、木材や木材複合材を含む多くの材料の品質を向上させるために使用できます。
補強材としてのナノセルロース
マトリックス材料の補強材としてナノセルロースを使用するという原則により、多くの研究が行われてきました。ナノスケールのセルロースを添加することにより、ナノコンポジットは、マイクロコンポジットでは達成できなかった多くの点で優れた特性を備えています[83]。これらのナノセルロース強化複合材料は、従来の複合材料を置き換えることができます。 NCCを適切に変更することにより、優れた特性または大幅に改善された物理的、化学的、生物学的、および電子的特性を備えたさまざまな機能性ナノ材料を開発できます。ナノコンポジットの特性は、マトリックス材料の特性、ナノセルロースの特性、マトリックス材料へのナノセルロースの分散、フィラーとマトリックス材料間の界面相互作用など、いくつかの要因に依存します[84]。
さまざまな種類のマトリックス材料への補強材としてのナノセルロースが広く研究されてきました。ナノセルロースで強化された天然または合成ポリマーからなるポリマーナノコンポジットは、有望なクラスの材料としての地位を確立しています。機械的特性の改善は、これらのナノセルロース強化ポリマーを調製する際に目標とする最も一般的な目的です[85,86,87]。石油ベースのポリマーは、一般的に熱可塑性と熱硬化性のタイプに分けられます。熱可塑性ポリマーと熱硬化性ポリマーの違いは、長鎖分子を保持する結合です。前者は弱いファンデルワールス結合によって保持され、後者は強い共有結合によって保持されます[88]。ナノセルロース複合材料で使用するために、さまざまな熱硬化性樹脂が研究されてきました。たとえば、エポキシ樹脂は、その優れた接着特性と硬化後の優れた機械的特性(高弾性率、低クリープ、および適度な高温性能)により、高度な材料製品に使用されています。ただし、高度に架橋された構造のため、衝撃で簡単に破損する可能性があります[89]。強化材料として官能化ナノセルロースを追加することで、エポキシベースのセルロースナノコンポジットの機械的特性が大幅に向上しました[90、91]。配向構造を持つエポキシ-セルロースナノファイバー複合材料を調製するためのアプローチが実施されています[92]。このプロセスは、バイオエポキシ樹脂を含浸させるためのプリフォームとして使用される前に、高度に多孔性のナノセルロースネットワークを調製するために、氷テンプレート(または凍結鋳造)法を組み合わせたものです。結果は、ナノコンポジットの弾性および貯蔵弾性率が、両方の試験方向で純粋なエポキシのものよりも優れており、強度が縦方向で改善されたことを示した。もう1つの最も一般的に使用される熱硬化性樹脂は、不飽和ポリエステル(UP)です。ナノセルロース含有量が45vol%と高く、これまでのどの研究よりもはるかに高いナノ構造UPバイオコンポジットは、正常に処理および特性評価されました[93]。ナノ構造のナノセルロースネットワーク補強により、UPの弾性率と強度だけでなく、延性と靭性も大幅に向上します。図2は、電界放出型走査電子顕微鏡(FE- SEM)。 FE-SEM画像は、アセチル化NCCがNCCと比較してPHBに均一に分散していることを明確に示しています。均質性は、強化材とポリマーマトリックス間の強力な界面相互作用に寄与しています[94]。
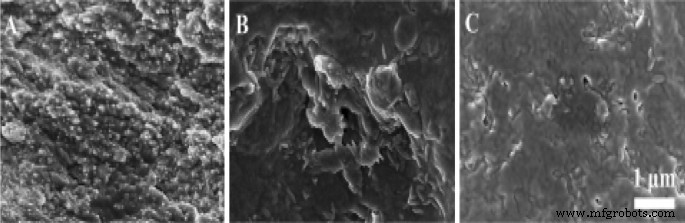
a の破壊形態のFE-SEM画像 PHB / NCC-15、 b PHB /アセチル化NCC(II)-15、 c PHB /アセチル化NCC(IV)-15ナノコンポジット。 ソース :Gan etal。 [94]。 ACSの許可を得て複製
表2は、PLA、PVA、デンプン、PU、PPなどの熱可塑性ポリマーへのナノセルロースの組み込みを示しています。これらのナノコンポジットの開発に利用されるプロセスには、とりわけ、溶媒交換、水性分散液、溶液キャスティング、グラフト化、コアバックフォーム射出成形、エレクトロスピニング、凝固および熱圧縮、およびその場でのアニオン開環重合反応が含まれる。ほとんどの研究では、ナノコンポジットにナノセルロースを添加すると、機械的特性(強度、剛性、耐クリープ性、弾性)、熱安定性、バリア特性、さらにはコンポーネントに応じてナノコンポジットの抗菌および抗酸化機能が改善されることが報告されています。使用されるナノコンポジットの。全体的な調査結果は、熱可塑性マトリックス中のナノセルロースの強化に関する肯定的な結果を報告しました。これは、より環境に配慮した持続可能な複合材料の製造に関するさらなる研究を促し、奨励します。
<図> 図>天然高分子は通常自然界に存在し、抽出することができます。天然高分子は、いくつかの例外を除いて、熱可塑性ではありません。ただし、化学的および物理的修飾技術は、セルロース、リグニン、キチンなどのバイオマス資源からの天然高分子の熱可塑性を誘発することができます。環境への意識の高まりとグリーン製品の需要により、ナノセルロースで強化されたさまざまな天然高分子がバイオナノコンポジットの製造に使用されてきました。骨組織の再生における植物由来のナノセルロースの使用を報告している研究は非常に限られています。研究の1つ[113]は、ヒドロキシアパタイト(HA)を使用して(TEMPO)酸化ナノフィブリル化セルロース(TNFC)またはナノ結晶セルロース(NCC)を合成することによるナノコンポジットの調製を報告しました。複合材料は、NCCベースのものよりも、外側および高密度の皮質骨の範囲で、より優れた圧縮強度、弾性率、および破壊靭性を示すことがわかりました。さらに、複合材料は、ヒトの骨由来骨芽細胞に対して細胞毒性を誘発しませんでしたが、むしろそれらの生存率を改善し、耐荷重用途での骨組織再生に有望です。
使用される他の天然生体高分子の中には、アルギン酸ナトリウム、セルロース、および生分解性、生体適合性、低毒性などの特徴的な特性を有するタンパク質があります。たとえば、アルギン酸ナトリウムは、組織工学、ドラッグデリバリー、食品包装、生物医学的応用などの多くの分野で優れた生体材料として広く使用されています。ただし、機械的強度が低く、劣化特性が制御されていないため、その用途が制限されます。アルギン酸塩マトリックスにナノセルロースを組み込んだナノコンポジットフィルムを開発することにより、これらの問題を克服するためにいくつかの試みが行われてきました。 NFCをアルギン酸塩マトリックスに組み込むと、耐水性と機械的特性が向上することがわかりました。さらなる調査により、分散を促進するための超音波処理により、TEMPOを介した酸化NCCは、NFCと比較してアルギン酸生体高分子の強化効率が高いことが示されています。ナノセルロースをキチン、キトサン、大豆タンパク質分離物(SPI)、亜麻仁ガムマトリックスに統合する際のバイオインスパイアード相乗的強化戦略の同様の概念は、高性能ナノコンポジットを構築するための新しい道を開きます。
木材ベースのパネル特性強化のためのナノ粒子
木材複合材は、一般に、バインダーによって一緒に保持された木材要素の組み合わせを有する広範囲の製品として説明されます。木材複合材の利点の中には、さまざまな厚さ、グレード、サイズで特定の品質や性能要件に合わせて設計できることがあります。木材複合材は、木材の自然な強度特性を利用するように製造されています(通常の木材よりも構造強度と安定性が高くなる場合があります)。他方、木材複合材には、固形材と比較した場合、製造に多くの一次エネルギーを必要とするという欠点もあります。したがって、木材複合材は、水を吸収する可能性があり、無垢材よりも湿気による反りが発生しやすいため、屋外での使用には適していません。使用した接着剤は、完成品に有毒なホルムアルデヒドを放出します。ナノテクノロジーを利用して、木質複合材料の品質を向上させ、既存の製品や新しいアプリケーションで使用される新製品に対する需要の高まりに対応できます。
木材の主な欠点は、微生物による感受性と生分解性、およびさまざまな含水率にさらされたときの寸法の不安定性です。これらは主に、細胞壁の主要ポリマーとそれらの豊富なヒドロキシル基(OH)によるものです[114]。木材は自然に吸湿性であり、木材による吸湿は露出表面積に直接関係しています。木材複合材に無機ナノ粒子を添加すると、複合材の抗菌特性が向上することが報告されています。酸化亜鉛(ZnO)のナノ粒子は、優れた抗菌活性を示します。これらのナノ粒子は、パーティクルボードの製造に使用される前に、メラミン-尿素ホルムアルデヒド(MUF)接着剤に添加されます[115]。調査結果は、グラム陽性菌黄色ブドウ球菌に対するパーティクルボードの生物学的耐性が増加したことを示しています。 、グラム陰性菌 Escherichia coli 、カビ Aspergillus niger および Penicillium brevicompactum だけでなく、茶色の腐敗菌 Coniophora puteana 。よく知られている殺生物剤添加剤である銀ナノ粒子も、パーティクルボードのメラミンラミネート表面に適用した場合、同様の抗菌効果とカビ防止効果を示しました[116]。ナノ銅酸化物とアルカン界面活性剤の組み合わせも、処理された合板標本の耐水性と耐シロアリ性を改善することが確認されました[117]。パーティクルボードの耐腐朽性を高めるための別のオプションとして、加工デンプンベースの接着剤が検討されました。ナノシリコンオキシド(SiO 2 )そしてホウ酸は、それらの天然澱粉と結合したパーティクルボードよりも腐敗しにくいことがわかった[118]。ナノSiO 2 の追加 撥水剤および抗真菌剤としてのホウ酸は、それぞれ、最終的なパーティクルボードでの微生物の活動を妨げています。
木材複合パネルの製造は、ホットプレス中の樹脂の硬化時間を短縮する方法を開発することによって即興で行うことができます。これにより、製造がスピードアップしたり、ボードの全体的な品質が向上したりする可能性があります。木材複合パネルのプレス時間に影響を与える熱伝達は、厚さ、プレス温度、閉鎖速度、およびマットの水分分布によって異なります。 ZnOナノ粒子の添加により、ホットプレス中のパーティクルボードの中心での熱伝達が増加し、樹脂の硬化度が高まり、物理機械的特性が向上しました[119]。多層カーボンナノチューブ(CNTS)や酸化アルミニウム(Al 2 )などの高導電性ナノ粒子 O 3 )は、中密度繊維板の熱的および機械的特性を向上させることも証明されています[120]。この研究では、活性炭ナノ粒子はボードの物理的および機械的特性に大きな影響を与えませんでしたが、他の2つのナノフィラーと比較して、尿素ホルムアルデヒド(UF)の硬化とホルムアルデヒド放出の低減に対してより加速された効果があることも報告されました。 。
木材複合材料の製造において、接着剤は非常に重要な役割を果たし、機械的特性、湿潤状態での性能、環境への影響など、複合材料の特性に影響を与えます。尿素ホルムアルデヒド、メラミン尿素ホルムアルデヒド、フェノールホルムアルデヒドは、木材複合材業界で一般的に使用されています。ナノ粒子の利用により、接着剤の特性が向上しました。物理的および機械的性能が向上し、ホルムアルデヒドの放出が低減されたナノ材料強化木材複合材料を製造するために、多くの研究が行われてきました。ナノクレイは、樹脂マトリックスの優れたフィラーと補強材であり、強度、靭性、その他の特性を大幅に向上させることが示されています。急速に成長するプランテーションからの3つの森林種で製造された合板用のナノクレイ粒子を使用したUF接着剤の変更: Cordia alliodora 、 Gmelina arborea および Vochysia ferruginea MuñozとMoyaによって報告されました[121]。 0.75%のナノクレイで樹脂をナノ修飾すると、ボードの破壊係数と弾性が改善されることがわかりました。 PF接着剤にナノクレイ粒子を使用する効果は、ボンドラインの接着剤の機械的特性を大幅に向上させ、合板のマクロ接着強度の向上に貢献しました[122]。興味深いことに、遷移金属イオン修飾ベントナイト(TMI-BNT)ナノクレイを使用して、insituインターカレーション法によって水素結合をブロックすることにより結晶性UF樹脂をアモルファスポリマーに変換しました[123]。これにより、接着強度が56.4%増加し、ホルムアルデヒド放出が48.3%減少しました。
木材複合材の物理的および機械的特性を即興するために使用される他のナノ粒子には、ナノウォラストナイト(NW)[124、125]ナノZnO [126]、ナノSiO 2 、nano-Al 2 O 3 [127]、ナノシルバー[128]およびナノセルロース(NCCおよびNFC)。 NCCは接着剤のフィラーとして使用されましたが、NFCは複合ボードの配合にバインダーとして適用されました。最近、セルロースのマイクロファイバーとナノファイバーの添加が樹脂の特性に有利な効果をもたらすことが明らかになりました。調査に基づいて、NCCを追加すると、固体樹脂100 gあたり10%の量で合板の機械的特性が大幅に向上することがわかりました[129]。一方、UF接着剤にNCCを添加した後のパーティクルボードの物理的特性は、滑らかな表面、パネルの密度と含水率のわずかな違い、およびナノセルロース含有量の高い値のみが厚さの膨潤において有意に高いことを示しました[130]。一方、接着剤としてNFCを使用して製造されたパーティクルボードパネルは、低密度グレードの機械的特性に関する業界の要件を満たすことが示されました[131]。高密度パーティクルボードの場合、NFC比の増加と圧力の上昇により、内部結合特性が向上する可能性があると推定されました。 NFCの添加率とパネル密度の増加に伴い、釘とフェーススクリューの引き抜き強度も増加することがわかりました[132、133]。
ウッドコーティング
森林は、主にまたは部分的に木材および非木材林産物の生産に使用されます。非木材林産物には、竹、籐、薪、木炭、ダマール、ヤシなどがあります。高品質の木材に対する需要は非常に高いですが、天然林からの木材の入手可能性は低下しています。その結果、木材の代替としての非木材資源の探索が加速されました。その急速な成長特性のために、竹は最も重要な非木材林産物の1つに発展しました。木材は、生物学的に自己組織化された天然の高分子構造(セルロース、リグニン、ヘミセルロース)です。これは最も用途の広い材料の1つであり、何世紀にもわたって建物や構造物の形で使用されてきました。しかし、木材は、光酸化、化学酸化、熱分解、紫外線(UV)光、水分、化学汚染物質、熱/冷気の変化などの環境からの光分解反応などの激しい酸化分解プロセスにさらされます[134]。竹自体のような非木材材料でさえ、タンパク質、炭水化物、その他の栄養素が豊富で、蛾に食べられて腐敗し、カビが発生しやすい天然有機材料です。したがって、木材および非木材製品の最終製品は、従来、保護および美的外観の改善のためのコーティングとして使用できる添加剤、火災および生物学的要因(真菌および昆虫)に対する保護のための防腐剤を含む。
コーティングは、基板表面に層を適用するプロセスです。木材の表面に塗布される一般的なコーティングの例は、ワニス、ラッカー、および塗料であり、その目的は保護と装飾の両方になります。コーティングの主成分は、バインダー、顔料、溶剤、フィラー、添加剤などの基本的な特性を決定します[135]。各要素は、木材の表面に特定の特性をもたらします。バインダーは、木材への顔料の接着に寄与し、保護層を作成しますが、顔料は色を提供し、非半透明の表面層を形成します。溶剤はコーティング用途に必要な粘度を与え、フィラーの添加はコーティングの色の濃さと光沢を変えます。添加剤に関しては、カビや腐敗を防ぎ、乾燥プロセスを助け、接着性を改善し、仕上げを制御します。ただし、柔軟性の制限、強度の低下、コーティング層と基板間の不均衡な接着、耐摩耗性の低下、耐久性の低下など、コーティングには弱点があります。
ナノコーティングには、これらの問題を解決する機能があります。ナノコーティングは、いくつかの特性を改善したり、新しい機能を付与したりするために、約<100nmの厚さの薄層を基板上に堆積させるプロセスです。ナノコーティングは、ナノ材料だけでなく、基板表面のトポグラフィーに影響を与えることなく、非常に薄い層のコーティングを施したかさばる材料にも使用できます。木材および木材製品へのナノコーティングの適用は、主に耐久性、機械的特性、耐火性、UV吸収の改善、および吸水率の低下に焦点を当てています。ナノコーティングの機能性とエンドユーザーの価値を高めるための1つのアプローチは、ナノ粒子の追加です[136]。これらのナノ粒子は、その形態のために表面積対体積比が非常に大きく、周囲と集中的に相互作用することができ、ナノサイズによって透明性が維持されます。
耐久性向上のためのナノ添加剤
ナノコーティングは、製品の分子レベルで変化をもたらすナノ粒子とナノデリバリーシステムを利用することにより、木材および非木材製品の耐久性を向上させることができます。コーティングの目的の1つは、真菌や細菌などのさまざまな微生物の増殖を防ぐことです。酸化亜鉛(ZnO)[137、138]、酸化チタン[138、139]、酸化セリウム(CeO 2 などの金属酸化物のナノサイズ粒子 )[140]は強力な抗菌特性を示すことが報告されました。ナノ粒子を木材表面に直接堆積させるか、ナノ粒子で木材表面を直接機能化する研究が行われています。 ZnOナノ粒子は、ゾルゲル法で調製されたZnOシード層に基づく単純な低温湿式化学法によって竹材の表面にうまく製造されました。調査結果は、処理された竹材が Aspergillus niger V. Tiegh に対してより優れた耐性を持っていることを示しました。 (A.ニジェール)および Penicillium citrinum Thom (P. citrinum)、しかし Trichoderma viridePersに対する耐性は低い。 ex Fr (T. viride)[141]。グラフェンは、細菌の増殖を抑制する優れた能力も示しています。したがって、還元型酸化グラフェンとナノZnOを利用して、竹ベースの屋外材料を2段階の浸漬乾燥および水熱プロセスでコーティングすることにより、耐カビ性と抗菌活性が向上します[142]。同様に、水熱プロセスを使用したナノ構造のZnOも、木材表面を生物劣化から効果的に保護します[143]。
ナノ結晶セルロース(NCC)と銀ナノ粒子(AgNPs)を組み込んだ水性ポリウレタン[144]コーティング(WPU)を使用して、木板の抗菌特性を改善しました[145]。 AgNPは抗菌材料で知られていましたが、準備プロセス中に簡単に凝集しました。したがって、NCCは、AgNPとWPUまたは他のコーティングとの混合および分散性を支援するために導入されました。さらに、NCCはナノコンポジットの機械的特性を改善するための優れた補強剤でもありました。ミニ乳化重合は、AgNPを含むアクリルラテックスコーティングの合成にも使用されました。これにより、木材表面での黒染み菌の増殖が制限されます[146]。パーティクルボードや中密度繊維板などの市販の木製複合材の処理中に適用されたアクリルコーティング中の銀および酸化亜鉛ナノ粒子の抗菌効果の研究が実施されました[147]。 AgおよびZnOナノ粒子は、グラム陰性菌 Escherichia coli に対して部分的により効果的でした。 グラム陽性菌黄色ブドウ球菌と比較して 。
吸水率向上のためのナノ添加剤
木材は水や湿気の影響を受けやすいことはよく知られています。これは、細胞壁構成ポリマーの親水性とその毛細管多孔質構造によるものです。木材と水の相互作用は、木材の生分解、寸法の不安定性、および風化の加速につながります。木材の疎水性を改善するために使用されている従来の化学修飾がありますが、木材への水のアクセスはまだ完全に遅れているわけではありません[148]。さらに、処理プロセスで使用される化学物質は危険である可能性があります。したがって、ナノテクノロジーは、木材の改質と機能化の代替アプローチとして使用されます。ポリマーコーティングへのナノ粒子の組み込みは、木材表面の吸水率を改善するために使用されます。ナノ粒子をコーティングに統合するために、2つのアプローチ、すなわち溶液混合とその場での添加が利用されます[149]。最初のアプローチ(溶液混合)は、溶媒が木材の表面に分散する前にポリマーと結合する場合です。この物理的方法は、浸漬、ブラッシング、スプレーによって適用できます[150]。ウーら[151]は、ポプラ材の表面に、ZnOナノ粒子とステアリン酸で修飾された水性UVラッカー製品(WUV)を介して、最大158.4°の接触角で超疎水性コーティングが構築されたことを報告しました。 WUVと比較して、ステアリン酸亜鉛/水性UVラッカー超疎水性コーティング(ZnSt 2 / WUV)の方が強力で、環境にやさしい超疎水性コーティングを作成するのに役立ちました。興味深いことに、TiO 2 を添加した水性ワニス ナノ粒子は、9つの熱帯木材種の仕上げを評価するために使用されました[152]。 TiO 2 の取り込みが見出された ナノ粒子は吸水率の値を低下させ、1年間の風化暴露後、TiO 2 を添加していないワニス ナノ粒子は完全に分解しましたが、改質ワニスフィルムは耐えました。うまく調製された超疎水性木材コーティングの他の例は、リグニンコーティングされたナノ結晶セルロース(L-NCC)粒子/ポリビニルアルコール(PVA)複合塗料システム[153]、UV光硬化性メタクリル-シロキサン-セルロース複合コーティング[154]、 Fe 3+ ドープされたSiO 2 / TiO 2 複合フィルム[155]とポリジメチルシロキサン(PDMS)/シリカハイブリッドコーティングシステム[156]。
2番目のアプローチは、モノマーへの化合物の直接添加とそれに続く重合を含む、その場での添加または化学プロセスです。ナノ粒子は、熱水法やゾルゲル法などの木材表面での化学反応によってその場で合成されます。 Gao etal。 [157]は、フルオロアルキルシランで修飾されたAgNPを使用して、超撥油性を備えた超疎水性導電性木材表面を調製するための簡単で効果的な方法を適用しました。多機能コーティングは、さまざまな用途、特にセルフクリーニングおよび生物医学電子デバイス向けに商品化することができます。別の研究では、竹をZnOゾルを使用して処理し、ZnOナノシートネットワークを竹の表面に熱水で成長させた後、フルオロアルキルシランで修飾しました[158]。処理に成功した竹は、堅牢な超疎水性、シミュレートされた酸性雨に対する安定した撥水性、UV耐性、耐火性などの優れた特性を示しました。アナターゼ型TiO 2 の熱水蒸着によって竹材を作成した場合にも、同様の優れた特性が得られました。 ナノ粒子であり、オクタデシルトリクロロシランでさらに修飾されています[159]。超疎水性の木材表面は、高分子電解質/ TiO 2 のレイヤーバイレイヤー[160]アセンブリなどのアプローチを使用して作成することもできます。 ナノ粒子多層およびパーフルオロアルキルトリエトキシシラン(POTS)[161]で疎水性修飾、水性パーフルオロアルキルメタクリルコポリマー(PMC)/ TiO2ナノコンポジットのPDMSプレコート基板へのスプレーコーティング[162]、およびテトラブチルチタネートを使用したワンステップ水熱プロセス( Ti(OC 4 H 9 ) 4 、TBOT)およびビニルトリエトキシシラン(CH 2 CHSi(OC 2 H 5 ) 3 、VTES)を共同前駆体として[163]。蓮の葉のようなSiO 2 を生成するための生体模倣アプローチでさえ ソフトリソグラフィーに基づく超疎水性竹表面が首尾よく実行された[122]。
機械的特性を改善するためのナノ添加剤
有機ポリマーに組み込まれた無機粒子は、機械的特性を高めるために木材コーティングで一般的に使用されます。フィラーとして、無機材料の剛性と硬度がポリマーの加工性と効果的に組み合わされています。無機粒子をミクロンサイズで塗布すると、材料の柔軟性が低下し、コーティングシステムの透明性が低下するなどの欠点があります[164]。ナノサイズの無機粒子を利用すると、表面積と界面面積の比率が増加し、その後、原材料の特性に影響を与えます[165]。最近の研究では、再生可能な補強材としてナノセルロースを使用して、性能が向上したバイオベースのナノコンポジットコーティングシステムを開発することを検討しています。ナノセルロースは、ポリマーマトリックスとの非相溶性の問題のために表面修飾されました。 TEMPO酸化セルロースナノファイバーの添加により、WPUコーティングの機械的特性が改善されました[144、166]。非極性ポリマーマトリックス[167]の場合、ナノ結晶セルロースは、塩化アクリロイルまたは陽イオン界面活性剤[167]の2つの方法で修飾されました。 NCC負荷レベルを最大2%増加させると、硬度、弾性率、および引張強度が増加します。
ナノシリカは、機械的特性の改善に適用されるもう1つの一般的なナノ粒子です。ナノシリカを使用する利点の中には、その高い硬度があり、ポリマーマトリックスとの適合性を改善するために化学的に容易に変更することができます。 Mengらによる最近の研究。 [168]報告されたヒマシ油ベースの水性アクリレート(CWA)/ SiO 2 有機-無機共有結合架橋ネットワーク構造を持つハイブリッドコーティングは、ゾルゲル反応とチオール-エン反応によって調製されました。この調査結果は、エマルジョンの安定性が良好であることに加えて、コーティングの熱的および機械的特性が10 wt%のSiO 2 で大幅に改善されたことを示しています。 。ナノシリカの添加による機械的特性の改善は、水性ニトロセルロース[169]やアクリレート[170]などの他のコーティングシステムでも説明されています。
UV吸収改善のためのナノ添加剤
木材の光分解のプロセスは、太陽光にさらされた直後に始まり、その後、色の変化と木材表面の進行性の侵食が発生します。紫外線は、木材のポリマー構造成分(リグニン、セルロース、ヘミセルロース)を光化学的に分解することができます[171]。光分解プロセスは通常、木材および木質材料の耐水性を低下させ、屋外暴露条件下でさらに生分解を引き起こします。太陽放射のUV成分による材料への激しい損傷は、光安定化技術、表面コーティングを使用するか、これらの材料をUV放射に対してより耐性のある材料に置き換えることによって防ぐことができます[172]。ナノ粒子を利用して、木材の表面を保護するために、溶剤、水性、およびUVコーティングのUV耐性を向上させることができます。 UV遮断特性を実現する機能性コーティングを含むナノ粒子は、表面の透明度に影響を与えることなく、UVに対する高レベルの保護を提供します。ナノ粒子のサイズが小さいため、表面積対体積比が大きいため、天然素材と比較して紫外線を遮断する効果が大幅に向上します。
紫外線吸収コーティングの使用は、紫外線によるリグニンの劣化を防ぐのに役立ちます。 UV吸収剤として使用されるナノ粒子には、主にTiO 2 があります。 およびZnO。 Wallenhorst etal。 [173]は、Zn / ZnOコーティングと追加のポリウレタンシーリングで構成されるシステムが、木材表面の光変色を大幅に低減し、化学的に安定していることを証明したと報告しました。ベンゾトリアゾール(BTZ)とZnOナノ粒子の組み合わせは、アクリルベースの竹の外装コーティングのUV吸収剤として適用されました[174]。特に2:1の比率の配合では、BTZ–ZnOコーティングで強力な相乗効果が検出されました。コーティングシステムは、光劣化に対する高い耐性を提供し、竹の下地の光変色を効果的に抑制しました。厚膜水性アクリルコーティング中のベンゾトリアゾール、ヒンダードアミン光安定剤(HALS)、およびZnOナノ粒子の別の混合物も、オーク材に適用した場合にUV保護表面改質に最も良い効果をもたらしました[175]。ベンゾトリアゾール、HALS、および両方のTiO 2 の混合物 ZnOナノ粒子は、UVおよびVISスペクトルによる木材の色安定化のための最も効果的な処理の1つとして確認されました。ルチルTiO 2 でコーティングされた木材標本が報告されました メチルトリメトキシシランとヘキサデシルトリメトキシシランの混合物は、優れた耐候性と、表面の色の変化および重量損失に対する改善された耐性を示しました[176]。 TiO 2 コーティングはまた、水を噴霧せずに紫外線を照射したときの木材の色の安定性を明らかに高めることがわかった。しかし、隣接する木材の表面は、TiO 2 の光触媒活性のために劣化しました。 [177]。
難燃性向上のためのナノ添加剤
木材および非木材製品の可燃性は利用を制限しており、火災の安全性はさまざまな用途の主要な関心事です。木材と非木材の固有の欠陥と使用を安全な方法で克服するには、難燃性を真剣に検討する必要があります。ナノ粒子は、難燃性を改善するためのナノコンポジットを製造するために最近使用されています。ナノ粒子を単独で、または従来の難燃剤と組み合わせて利用することで、木材の発火性を低下させることができます。ナノ材料のナノサイズと高表面積は、他の従来の化合物よりも低濃度でより効果的であり、産業的および経済的に大きな利点です。ナノ粒子がより適合性が高く均一な分散を実現するには、表面改質が必要です。 TiO 2 コーティングされた木材は、コーティングされていないサンプルと比較して、木材の可燃性と炎の広がりを減らすことができることがわかりました[178]。 ZnO–TiO 2 -層状の二重ナノ構造は、竹の基板上に合成されていました[179]。調査結果は、ZnO–TiO 2 で覆われた後、酸素指数が25.6%から30.2%に増加したことを示しました。 コーティングは、その難燃性の大幅な向上を明らかにしました。層状複水酸化物[180]は、大量の熱を吸収し、可燃性ガスの濃度を希釈し、分解プロセス中に有害な酸性ガスを吸収する可能性があります。したがって、それは優れた難燃剤です。八尾他[180]ナノマグネシウムアルミニウム層状複水酸化物(Mg–Al LDH)をその場でのワンステッププロセスで竹に適用し、総熱放出と総煙生成がそれらと比較してそれぞれ33.3%と88.9%減少することを発見しましたMg–AlLDHを含まないサンプルの分析。王ら。 [181]は、亜鉛-アルミニウム層状複水酸化物(Zn-Al LDH)ナノ構造を木材に導入し、ピーク熱放出率(PHRR)と総煙生成がそれぞれ55%と47%減少したことを発見しました。手付かずの木材[181]。グラフェンなどのナノ構造炭素材料は、火災に対する表面保護のための木材および木材複合材料の効果的な難燃剤として使用される可能性が高いことも証明されました[182]。
木材の耐久性
木材は、建設、家具、アートワークなどのさまざまな分野で使用される非常に用途の広い素材です[183,184,185]。木材は、強度と重量の比率が高く、環境にやさしい特性、美的外観、生分解性を備えているため、建設資材として使用されています。残念ながら、木材は、特に腐朽菌や昆虫による生物攻撃に非常に敏感です[186、187]。木材は、紫外線、火、湿気にさらされることによっても影響を受けます[188]。湿気は、木材の反り、ひび割れ、寸法の不安定を引き起こす可能性があります。木材の劣化は、美的および内部の構造的損傷を引き起こす可能性があります。木材の劣化は、毎年計り知れない損失につながります。さらに、木材構造での腐朽菌の増殖は、特に長時間の屋内曝露後のアレルギー、呼吸器症状、喘息などの健康問題を人間に引き起こします[189]。したがって、木材に関連する問題は単純に見落とすことはできません。化学薬品を使用した木材の保存は、木材の耐用年数を保護および延長するための効果的な方法です。
過去数十年にわたって、多くの化学防腐剤が開発され、生分解剤から木材を保護するために使用されてきました[188、190]。残念ながら、これらの化学防腐剤のほとんどは、土壌や生態系に蓄積するため、人間、生物、環境に深刻な影響を与える可能性があります[191]。クロム銅ヒ素(CCA)は、1930年代半ばから広く使用されている化学防腐剤のひとつであり、腐敗した真菌、シロアリ、虫食い虫から木材を効果的に保護します。しかし、CCAは人と環境に有毒であることが示されました[192,193,194]。同様の問題は、別の化学物質、つまりペンタクロロフェノール(PCP)によっても直面しています。それは人間の健康に有害であると考えられていました。したがって、その生産と使用は多くの国で禁止されました[195]。
これらの問題に対応して、安全で汚染が少ないと主張されている新しい一連の化学防腐剤が導入されています。これらの化学防腐剤は、植物抽出物から得るか、合成的に製造することができ、植物の生物活性化合物の特性を持っています。たとえば、ピレスロイドの花( Chrysanthemum cinerariifolium )に由来するピレスロイド )、強力な殺虫活性を持ち、今では合成的に生産することができます。残念ながら、これらの化学物質は光と温度によって容易に分解されることがわかり、効率スペクトルが狭い[196]。有機農薬のその他の制限は、それらのほとんどが有機溶剤に溶解し、水性木材防腐剤として配合できないことです。したがって、スマートでインテリジェントな有機農薬デリバリーシステムが重要です。スマートデリバリーシステムにより、殺生物剤は制御されたターゲットを絞った方法でデリバリーできます。これにより、人と環境への危険が減少します[17]。
ナノテクノロジー法による木材処理は、生分解剤や天候に対する木材の耐久性を向上させることができます[14、197、198]。ナノ製品を木材に適用する主な利点は、木材構造に深く浸透する独自の能力であり[14、199]、耐久性が向上し、長寿命のサービスが得られます。一方、ナノ製品の均一な分布による完全な浸透も達成できます[200]。現在まで、木材保護用のすぐに使用できる多くのナノ製品(ナノ防腐剤)が市場に出回っていますが、そのうちのいくつかはまだ研究開発段階にあります。一般に、木材を保護するために使用されるナノ製品は、ナノカプセルとナノ材料の2つのタイプに分類できます。ナノカプセルとは、高分子ナノキャリアに埋め込まれた農薬を指し、ナノマテリアルは、木材に直接含浸できるナノサイズの金属です[201]。
ナノカプセル化による殺生物剤強化特性
農薬を高分子ナノキャリアにカプセル化することは、木材への農薬の含浸を改善する上で有望なナノテクノロジー技術の1つです。この技術は、難水溶性農薬の溶解度を高め、農薬をゆっくりと放出するように設計されています[202]。カプセル化により、これらの低溶解性農薬は固体高分子ナノ粒子に容易に分散します。次に、ポリマーを水に懸濁し、従来の水ベースの処理を介して木材に塗布することができます[149]。カプセル化は、親水性有効成分を過度の浸出から保護することもできます[19]。カプセルの直径が小さいため、木材の細胞壁に簡単に組み込んで浸透させることができます。したがって、これにより、生分解剤に対する処理木材の耐久性が向上します。この技術は農薬を安全に届けることができるだけでなく、農薬の寿命を延ばすことができ、その結果、木材の保護が延長されます[203]。
農薬のカプセル化はボトムアップアプローチです。これは、ナノ沈殿[17、204、205、206]、エマルジョン拡散[207]、二重乳化[208]などのいくつかの手法で実行できます(表3)。 The materials used as polymeric nanocarriers can be derived from natural polymer, synthetic polymer or combination of both [209]. For instance, natural polymer includes cellulose, starch, alginates, silica and halloysite. The synthetic polymer usually used as polymeric nanocarriers includes poly(vinyl acetate), poly(methyl methacrylate), poly(lactic acid), etc. (Table 3).
<図> 図>A previous study by Liu et al. [18, 216] successfully encapsulated tebuconazole and chlorothalonil into polyvinylpyridine and polyvinylpyridine-co-styrene using the impregnation method. The particle size of capsules obtained was between 100 and 250 nm. They impregnated a suspension of the capsules into sapwood of southern yellow pine and birch using conventional pressure treatments. The treated wood was then exposed to brown-rot (Gloeophyllum trabeum ) and white-rot (Trametes versicolour ) fungi for 55 days. The results showed great resistance against both decay fungi.
Salma et al. [17] used the nanoprecipitation technique to encapsulate tebuconazole. The polymer capsules containing tebuconazole were prepared from amphiphilic copolymers of gelatine grafted with methyl methacrylate with the size diameters ranging from 200 to 400 nm or 10 to 100 nm depending on core/polymer shell mass ratio. The encapsulated tebuconazole was reported to be able to preserve wood against a brown rot fungus. On the other hand, the formulation system developed by Salma et al. [17] is flexible and can be easily modified using copolymerisation of other acrylic monomers like hydroxyethyl methacrylate. This indicates that tebuconazole release rate can be tailor-made. However, the disadvantage of this nanocapsules is that they are prone to aggregation, which reduces the delivery efficiency of the nanocapsules into the wood.
Can et al. [217] successfully encapsulated nano silver into polystyrene-soybean co-polymer. In their study, the Scots pine was impregnated with the capsules and tested against white-rot fungi (Trametes versicolor )。 The finding obtained from the study indicates that the soybean oil, polystyrene and nanosilver played important roles in the synergistic effect of increasing the decay resistance of Scots pine.
Impregnation of Metallic Nanoparticles for Durability Enhancement
Metallic nanoparticles have been used to protect wood against biodegradation agents and weathering since decades ago [197, 218, 219]. Nanoparticles offer better characteristics than their bulk form, mainly because of their reduced size that leads to high specific surface area-to-volume ratio, uniform size distribution and good stability. Due to its very small size, nanoparticles can penetrate deeply and uniformly into the wood pores leading to a protection of wood [197, 220]. In addition, dispersion stability is improved because of the size and also by addition of surfactant [221]. Dispersion stability coupled with small particle size may greatly improve the following aspects:(1) preservative penetration, (2) treatability of wood, (3) stability of finishes and coating products, (4) low viscosity, and (5) non-leachability [222, 223]. Besides, it can enhance compatibility with binders thereby being able to increase the affinity with wood polymers [197, 224].
Metallic nanoparticle can be prepared by altering the particulate size of metal via chemical reactions, mechanical treatment, heating or refluxing. To date, a lot of nanoparticles have been used for wood protection. Metallic nanoparticles mainly copper, silver, boron and zinc exhibited a good performance against white-rot and brown-rot but less efficient against mould [219].二酸化チタン(TiO 2 ) is another nanoparticle that has a good potential to be used as wood preservative. The potential of TiO2 is related to its antibacterial and antifungal [14, 225] and UV-resistant properties [225, 226]. However, the study on wood treated with TiO2 is still under preliminary investigation [14, 225]. Table 4 lists the works on the utilisation of TiO2 for wood preservation. The distribution of TiO2 in wood was studied by Mohammadnia-Afrouzi et al. (Fig. 3).
<図> 図>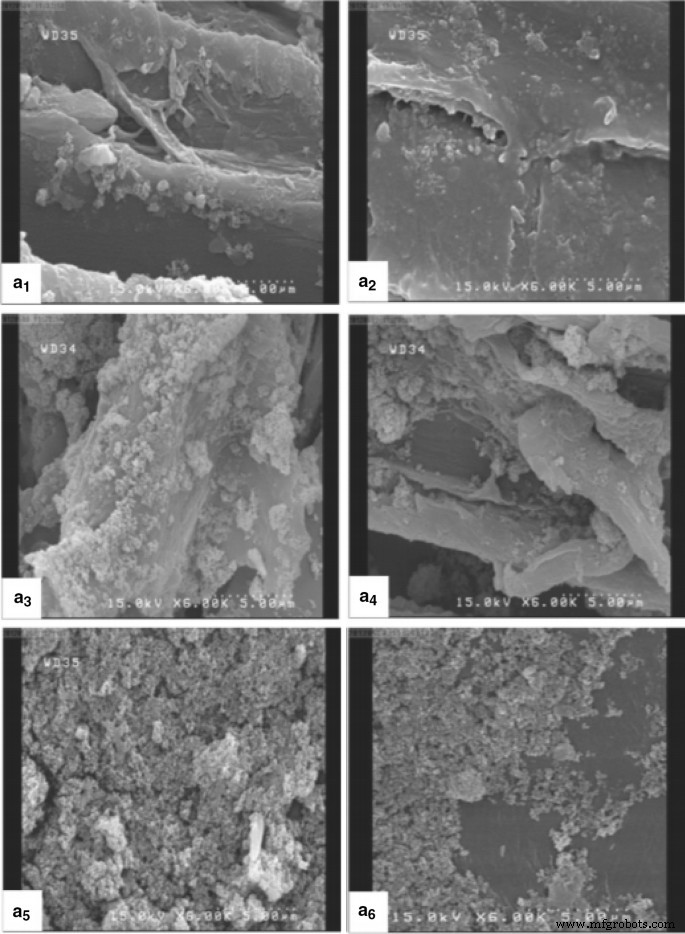
SEM images taken from the tangential section of nano-TiO2 wood treated samples:the 0% moisture content (MC)/0.5% concentration (a1), the 25% MC/0.5% concentration (A2), the 0% MC/1% concentration (A3), the 25% MC/1% concentration (A4), the 0% MC/1.5% concentration (A5) and (25% MC/1.5% concentration. Source :Afrouzi et al. [227]
Copper is an essential biocide for wood protection. However, copper alone fails to protect wood against copper-tolerant wood destroying fungi. Copper nanoparticles is a new generation of wood preservative-based copper. The use of copper nanoparticles instead of conventional copper shows improved durability of wood against decay fungi [230]. This shows that copper nanoparticles can be used to protect wood without the presence of chromium and arsenic [230].
Cristea et al. [15] studied the effects of the addition of ZnO nanoparticles and silver nanoparticles into exterior wood coatings. The purpose of the study is to improve the durability and wood protection through UV shielding. Besides providing an efficient protection against UV, the mechanical properties of wood such as hardness, adhesion strength, the abrasion resistance and the barrier effect for water vapor diffusion were slightly improved.
The mixture of ZnO nanoparticles with silver nanoparticles was able to protect wood from weathering problem such as UV rays [231]. They impregnated sapwood of cottonwood using three different concentrations of mixture by full-cell process. The samples were then exposed to natural weathering. The colour changes of treated wood samples were measured using spectrophotometer. The wood treated with ZnO nanoparticles alone was used as a control. The finding indicates that wood treated with the mixture of ZnO nanoparticle and silver nanoparticles has the lowest colour changes compared to the samples treated with each metallic nanoparticles.
In another study, Mantanis et al. [197] treated black pine wood with ZnO, zinc borate and copper oxide nanoparticles under vacuum. They used acrylic emulsion to force the metallic nanoparticles into the wood structure to avoid leaching. The durability of treated wood against mould decay fungi and subterranean termites was evaluated. Results showed that wood treated with zinc borate slightly inhibited the mold, while the other metallic nanoparticles did not exhibit mould. A similar finding was reported by Terzi et al. [232] that ZnO nanoparticles did not exhibit the mould growth on wood specimens. However, all metallic nanoparticles significantly inhibited white-rot fungi and termite.
Another study shows that metallic nanoparticles are able to improve fire retardant properties of wood. Francĕs et al. [16] studied the effect of SiO2 、TiO 2 and ZnO2 infiltrated into pine veneers. They reported that veneer treated using 3 wt% of SiO2 was most effective to improve the fire retardant behaviour.
Despite the remarkable advantages of nanotechnology in the wood preservation sector as discussed above, the fundamental understanding on synthesis, processing and characterisation of nanocapsules and metallic nanoparticles for wood protection still needs to be improved. The most interesting characteristics need to be considered during the design and development of nanocapsules or metallic nanoparticles for wood protection are as listed in Table 5.
<図> 図>Potential of Nanocellulose-Based Material in Energy Sector
Energy is an important resource that has a strong correlation between economic growth and development [233]. Today, the main energy sources are from fossil fuels and hydroelectric sources, which are very harmful to environment because they can cause climate change and global warning as well as ozone layer depletion, pollution, greenhouse gases emission and ecological destruction [234,235,236]. About 80% of carbon dioxide (CO2 ) emissions in the world are from energy sector and technology advancement is required to develop sustainable renewable energy resources to reduce the CO2 emissions as well as to overcome the global warming impact on life and health in line with the needs of accelerating technology development [234, 237].
In order to minimise the environmental effects, a sustainable and low-cost energy efficient carbon-based material has been explored as a potential to replace some conventional materials in the fabrication of energy devices. One of the natural carbon-based materials is cellulose which is the most promising natural polymer with many usages, including energy [237].
Nanotechnology is one of the advanced technologies that have the potential and prospect to fulfil the demand to create clean and green energy. Developing this new material in nanoscale enables new application and its interaction with current energy technology that would revolutionise the energy field from usage to supply, conversion to storage and transmission to distribution [238]. By adapting this nanotechnology, it will have high impact on the development of clean and green energy and benefits the environment and natural resources [239].
According to Serrano et al. [234] and Hut et al. [235], most promising application of nanotechnology for energy conversion is mainly focused on solar energy, conductive materials, solar hydrogen, fuel cells, batteries, power generation and energy devices. Understanding the structural and morphological properties of nanomaterial is essential to obtain the proficiency and sustainability for many applications. The greatest application of nanotechnology in energy generation is solar energy using photovoltaic (PV) cells which focuses on harnessing efficiency [233]. Consumption of energy generated from this solar cell using natural resources will reduce the usage of fossil fuel and decrease the pollution towards creating environmentally friendly and green energy [239]. In addition, the development of nano devices using solar cell could improve the existing materials efficiency as well as reducing manufacturing cost that might increase the economic growth [236].
Nanotechnology has been used in various applications to improve the environment, to solve humanity problems and to produce more efficient and cost-effective energy, such as generating less pollution during products manufacturing, producing solar and fuel cells at a competitive cost, hydrogen production, cleaning up organic chemicals polluting groundwater and overcoming the problem of energy sufficiency, climate change and diseases as well as to reduce the dependency on non-renewable energy sources [233, 235, 240].
Nanocellulose-Based Material for Solar Energy
Solar energy is available in various parts of the world and can be captured from the sun with 15,000 times more energy yearly. This energy source can be used in different ways:photovoltaic (PV) technology, solar thermal systems, artificial photosynthesis, passive solar technologies and biomass technology, which are used to produce electricity, steam or biofuels [234, 235].
In future, nanotechnology might contribute to develop an effective and low-cost system for production, storage, and transporting of energy [235]. According to Serrano et al. [234] and Hut et al. [235], current photovoltaic (PV) market is based on silicon wafer-based solar cells (first generation) and thin film layers of semiconductor materials (second generation). Current drawback of using solar cells is the cost of manufacturing mainly on the high cost of conventional PV cells with poor energy absorption efficiency (less than 40%) [233].
Nanocellulose shows a good potential to be used in the solar energy system due to its renewability, biodegradability, biocompatibility, broad modification capacity, adaptability and versatile morphology [241, 242]. Low cost, flexible and porous substrate of cellulose could be used to produce solar cells. Nanofibrillated cellulose (NFC) with size as low as 4 nm could become the excellent candidate for production of ultrathin paper for use in solar cell component to store the energy [235]. Klochko et al. [243] used nanocellulose from biomass for the development of biodegradable eco-friendly flexible thin film as a thermoelectric material. The thin film was used to convert low-grade waste heat from sun radiation into electricity at near-room temperature.
Nanocellulose-Based Conductive Materials
Conductive materials allow the flow of electrical current which is needed in the fabrication of energy devices. There are many types of conductive materials such as conductive polymers and conductive carbon materials (e.g. carbon nanotubes, graphene, and carbon black) and metallic particle with different levels of conductivity. These conductive materials can be combined with nanocellulose to form novel composites. The process of production conductive nanocellulose is shown in Fig. 2. There are two major strategies involved in nanocellulose based conductive hybrids fabrication process; one is coating of conductive materials layer on the surface of nanocellulose substrates, and another one is mixing the conductive materials inside the nanocellulose substrate to make composite [237]. Conductive polymers are an alternative to metallic materials because of their good electrochemical performance, light in weight and low cost. One of the most promising conductive polymers is polyaniline (PANI) because of its simple route of synthesis, controllable conductivity and high specific capacitance [244].
Nanocellulose-based conductive materials are developed for supercapacitors and energy storage device applications using various types of method such as in situ polymerisation, doping, coating, inkjet printing and in situ depositing [68, 244,245,246]. Modification of the existing supercapacitor by adding nanocomponents has increased its ability to store large amount of energy with longer time of supply [238].
Besides that, nanocellulose-based composite membrane electrodes can be developed via in situ polymerisation of nanocellulose using conductive components via a simple filtration unit (Fig. 3). A well-mixed conductive materials/nanocellulose composite membrane is left on the filter after the liquid has passed through the filter and air-dried composite membrane can be peeled off from the filter membrane for further use as supercapacitors [244].
Nanocellulose for Energy Storage
The potential application of nanocellulose for energy storage application has gained much attention recently. This is due to its nanoscale dimension, high surface area-to-volume ratio, and rich with hydroxyl group, which make their surface chemistry easily modifiable for composite processing. The most important aspect in energy storage is to develop nanocellulose with conductivity and flexibility properties. It can be achieved by adding conducting polymers such as polyaniline and polypyrrole. For example, the nanocellulose/polyaniline composite film is widely used as paper based sensors, flexible electrode, and conducting adhesive [68, 247,248,249]. Razaq et al. [250] manufactured electrodes from the composite of nanocellulose/polypyrrole and carbon filament for paper based energy storage devices.王ら。 [248] reported that their devices which developed using composite of nanocellulose/polypyrrole provide high charge and discharge rate capabilities, high cell capacitances, and cycling performance.
Nanocellulose-Based Materials for Lithium and Vanadium Battery
High demand on flexible portable electronic devices recently such as smart phone, electrical vehicles, laptops, and even the grid energy storage causes increasing demand on rechargeable lithium-ion battery (LIBs) [251] and supercapacitors [252]. LIBs are one of the most ideal energy storage candidates for electronic devices, due to their high energy density, moderate power density and cycle stability. In LIBs, electrolyte is important for lithium-ion (Li + ) transfer between anode and cathode. Organic liquid electrolyte is used for LIBs system, but it can pose tremendous safety concern due to the high toxicity and flammability. Solid-state electrolyte has become of interest because it demonstrates obvious advantages of low flammability and low toxicity [253, 254]. According to Janek et al. [255], solid-state electrolyte is classified as solid polymer electrolyte (SPE) and inorganic solid electrolyte (ISE). However, SPE offers the advantages of easy processing and flexibility [255].
秦ら。 [256] developed SPE by incorporating polyethylene oxide (PEO) with nanofibrillated aerogel and bis(trifluoromethanesulphonyl)imide lithium salt (LiTFSI). The results showed that the ionic conductivity properties of SPE were significantly enhanced due to the negatively charged nanofibrillated cellulose. The results also proved that the fabricated SPE is electrochemically stable, mechanically robust and thermally stable as well as flexible, expected for use in flexible electronic devices.
Nair el at. [257] fabricated nanocellulose-laden composite polymer electrolyte for high performing lithium-sulphur batteries using a thermally induced polymerisation method. The composite polymer electrolyte demonstrates excellent ionic conductivity, thermal stability up to more than 200 °C and stable interface towards lithium. The electrolyte also has stable cycling profiles which are attributed to significant reduction of the migration of polysulphide towards anode by entrapment of nanocellulose in the polymer matrix.
Another study was carried out by Zhang et al. [258] on robust proton exchange membrane developed using sulphonated poly(ether sulphone) reinforced by core–shell nanocellulose for vanadium redox flow batteries (VRFBs). It was found that with the incorporation of silica–encapsulated nanocellulose, the proton exchange membrane exhibits outstanding mechanical strength of 54.5 MPa and high energy efficiency above 82% at 100 mA cm −2 , which is stable during 200 charge–discharge cycles. Proton exchange membrane is one of the key components in VRFBs. It functions as the separator to avoid vanadium ions crossover. It also acts as proton conductors that contribute to high voltage efficiency in VRFBs [259].
Nanocellulose-Based Materials for Flexible Supercapacitor
Supercapacitor or known as electrochemical double-layer capacitor or ultracapacitor is another versatile energy storage system that has gained the attention of researchers worldwide [260]. With the high power density, long life cycle, simple principles, low maintenance, portability, stable performance and fast charge/discharge rate make supercapacitors capable of filling the gap between batteries and conventional capacitors [260]. These properties offer a promising approach to meet the growing power demands.
Electrode is a very important component in supercapacitor. It requires good electrochemical performance and flexibility especially for preparing a high-performance flexible supercapacitor. Him et al. [261] and Zhe et al. [262] found that graphene and nanocellulose are excellent flexible electrode materials for supercapacitors. Nanocellulose has been used as a substrate material because of its good biodegradability, mechanical strength, flexibility, and chemical reactivity. The porous structure and hydrophilicity of nanocellulose can facilitate the attachment of other materials for example graphene in their fibrous network structure [263]. At the same time, the abundance of hydroxyl groups on the nanocellulose surface enables the interaction of nanocellulose with other polymer to form strong composites [264].
Khosrozadeh et al. [265] developed an electrode for supercapacitor using nanocellulose-based polyaniline/graphene/silver nanowire composite and after applying it for 2400 cycles, at a current density of 1.6 A/g, the supercapacitor showed a power density, energy density and capacitance of 108%, 98% and 84%, respectively. This shows that the electrode has an excellent cyclic stability and good mechanical flexibility. On the other hand, Ma et al. [266] developed an electrode using bacteria cellulose/polypyrrole coated with graphene. The prepared electrode has a good mechanical flexibility in which it can bend at any angle. The area capacitance and energy density can reach 790 mF cm −2 and 0.11 mWh cm −2 , respectively, when assembled into symmetric supercapacitors. The nanocellulose-graphene electrode can be fabricated using chemical cross-linking or physical cross-linking method [267, 268]. Nanocellulose as an electrode component of supercapacitor also plays the role of internal electrolyte reservoir. This is because nanocellulose can provide an effective way for ion transport. The high pore structure and hydrophilic properties of nanocellulose make it easier to transport electrolyte ion [261].
Nanocellulose-Based Paper for Electronic Devices
Nanocellulose-based paper is a green substrate that can be used for electronic and optoelectronic devices. Currently, commercial paper has relatively rough surface and weak mechanical properties which can be quite problematic for electronic device fabrication [269]. Most of the electronic device’s fabrication use non-biodegradable and non-recyclable component such as plastics, glasses and silicones as substrates [270]. Formation of nanocellulose-based paper from NFC via simple filtration method can produce mechanically strong and low coefficient thermal expansion paper as an alternative to commercial paper [6, 269]. According to Li and Lee [270], transparent nanocellulose-based paper for electronic devices has been designed and being applied for electrochromic, touch sensor, solar cells, transistors, organic light-emitting diodes (OLEDs), gravure printing proofer and radio-frequency identification (RFID).
One of the applications of transparent nanocellulose paper is for the production of flexible electronics materials through printing circuit directly on the surface of substrates via coating or thermal deposition techniques. Firstly, the fabrication of flexible electronics was built on special silicon wafer that allows the silicon nanomembranes (Si NMs) to be released and then transferred to NFC substrate with an adhesive layer, and the device was completed by photoresist patterning and dry etching steps as illustrated in Fig. 4 [271].
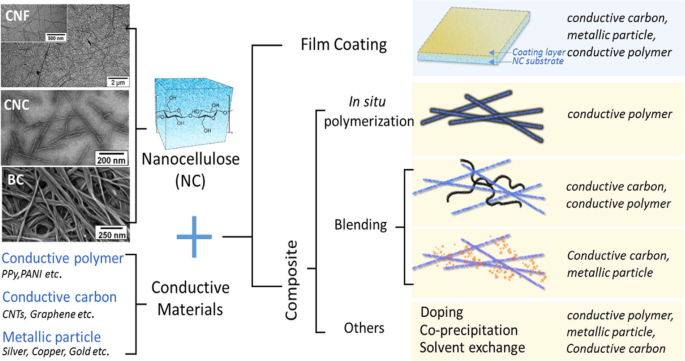
Schematic illustration of the generalised fabrication routes to nanocellulose-based conductive hybrid. ソース :Du et al. [237].エルゼビアの許可を得て複製
Moreover, NFC also is the main substrates for organic light-emitting diodes (OLEDs) (Fig. 5). Okahisa et al. [272] reported that the OLEDs device was fabricated on wood-based nano fibrillated cellulose (NFC) composite.
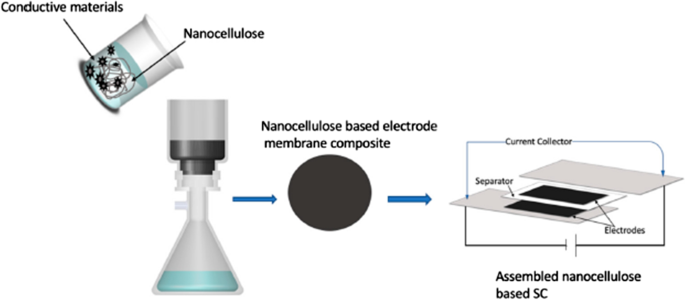
Filtration procedure to fabricate nanocellulose-based composite membrane [supercapacitor (SC)]. ソース :Hsu and Zhong [244]
Potential of Nanocellulose-Based Composite for Development of Sensor
Nanocellulose has been widely used to develop novel sensors and improve the sensitivity of sensors. In food industry for example, sensors have become an important tool to protect humans from health hazards and risks caused by the food contaminants. Sensors can help to quickly identify mycotoxins, pathogens, heavy metals, pesticides, metal ions, and so on in food. In addition, sensor technology can overcome the complicated, laborious, and time consuming process using expensive instrument that usually requires well-trained personnel [273, 274]. Sensor also provides rapid and sensitive food safety detection.
In the last decade, plenty of sensors (electrochemical sensors, biosensors and chemical sensors) have been successfully developed as alternative or as complementary detection tools for the rapid and sensitive detection [275,276,277]. However, conventional sensors were developed using plastics, petrochemical-based products and inorganic material which lead to environmental problems. Moreover, issues such as green gas emission, toxicity and sustainability of the materials are becoming increasing. Therefore, the demand for sustainable sensor devices has increased rapidly in recent years.
Nanocelluloses from plants and bacteria have shown promising potentials due to their excellent physical, thermal, mechanical, optical and physical properties, which are important for fabricating high-performance sensor devices. These properties make nanocellulose more preferred as biomaterials since it can enhance the selectivity and sensitivity of sensors for the detection of analytes. On the other hand, the adhesion properties of nanocellulose prevents the leaching problems of immobilised reagents. Thus a remarkable improvement can be obtained for the long-time stability of the sensor. Besides, the hydroxyl –OH groups on nanocellulose can be modified for the incorporation of binding sites for the selective adsorption of different analyte species [278]. The combination of those characteristics makes the nanostructured nanocellulose fibres an ideal building block for conjugation with other functional materials [248, 279, 280] (Table 6).
<図> 図>Development of Biosensors
キムら[286] immobilised enzymes candida rigosa lipase into the different cellulose nanocrystals. The findings indicate candida rigisa lipase absorbed on nanocellulose was relatively higher compared to that of microcellulose. This can be related to the high surface area on nanocellulose and thus increase the ionic interaction between nanocellulose anionic group and candida rigisa lipase. In this study, they found that the half-life of the candida rigisa lipase immobilised in nanocellulose increased 27 times higher compared to in free form. Besides, the stability of enzyme also increased (Fig. 6).
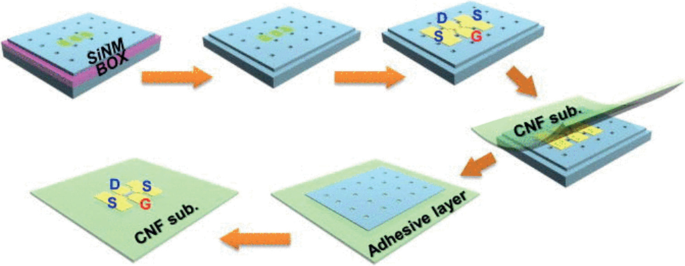
Flexible electronics production process on NFC substrate. ソース :Sabo et al. [271]
Another group of researchers, Edwards et al. [287] studied the kinetic profiles of tri- and tetrapeptides substrate of elastase for the fabrication of elastase biosensor whereby known as human neutrophil elastase (HNE) and porcine pancreatic elastase (PPE). To develop HNE and PPE, immobilised tri- and tetrapeptides were used into cotton cellulose nanocrystals. They found that 2 mg of tripeptide conjugated cotton cellulose nanocrystals in 1 h was able to detect 0.03 U mL −1 PPE, while 0.2 mL tetrapeptide conjugated cotton cellulose nanocrystals over 15 min could detect 0.05 U mL −1 HNE activity. Incani et al. [288] fabricated a biosensor by immobilising glucose oxidase (GOx) enzyme in a nanocellulose/polyethyleneimine (PEI)/gold nanparticles (AuNPs) nanocomposites. The AuNPs were adsorbed on the cationic PEI and nanocellulose. The Fourier Transform Infrared (FTIR) spectra confirmed that GOx was successfully immobilised on the polymer composites (Fig. 7).
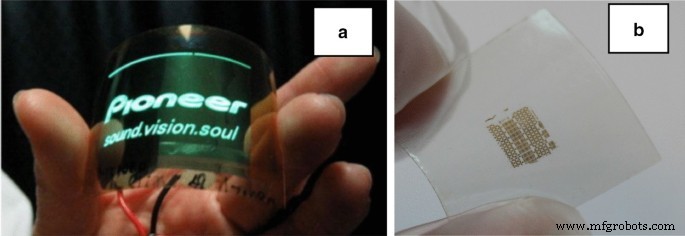
Flexible display (OLEDs) on NFC substrate, a ソース :Okahisa et al. [272]. Reproduced with permission of Elsevier. Flexible electronics on NFC substrate, b ソース :Sabo et al. [271]
Abd Manan et al. [289] successfully developed biosensor based on nanocrystalline cellulose (NCC)/cadmium sulphide (CdS) quantum dots (QDs) nanocomposites for phenol determination. They modified the NCC with cationic surfactant of cetyltrimethylammonium bromide (CTAB) and further decorated with 3-mercaptopropionic acid (MPA) capped CdS QDs as a scaffold for immobilisation of tyrosinase enzyme (Tyr). The TEM images of NCC and CTAB-NCC (Fig. 8a, b) indicates that agglomerated whiskers-like structure of NCC was not affected by modification, while the MPA QDs are of spherical shape (Fig. 8c). The FESEM images of CTAB-NCC nanostructured film (Fig. 8d) exhibited a homogenous, uniform and dense fibrous structures aggregated, while for CTAB-NCC/QDs nanocomposites film (Fig. 8e), the CdS QDs were appeared as like tiny white dots. EDX analysis shows the presence of respective elemental of carbon (C), oxygen (O), sulphur (S) and cadmium (Cd), indicating the CdS QDs was successfully attached into the CTAB-NCC film. The test finding against phenol shows the biosensor exhibits good linearity towards phenol in the concentration range of 5–40 µM (R2 = 0.9904) with sensitivity and limit of detection of 0.078 µA/µM and 0.082 µM, respectively.
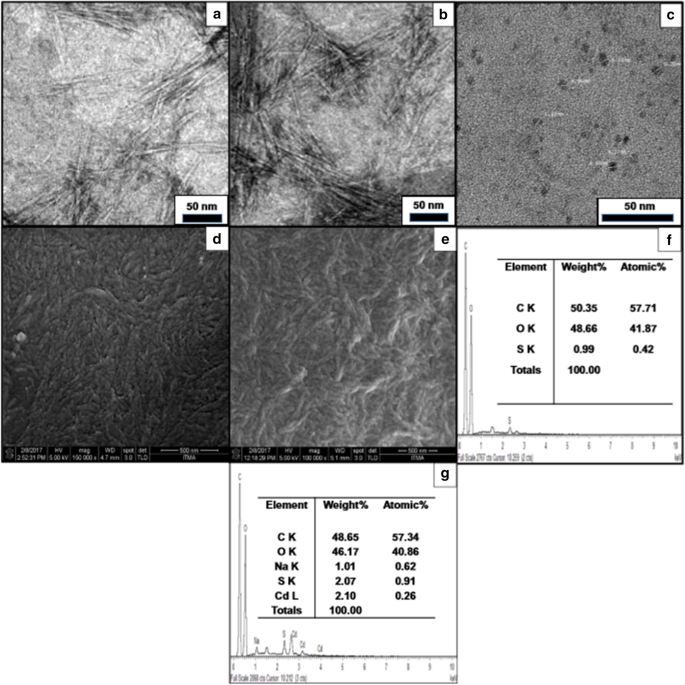
The TEM images for a NCC, b CTAB-NCC, c MPA-QDs, the FESEM micrograph of d CTAB-NCC nanostructures film, e CTAB-NCC/QDs nanocomposites film, f EDX analysis of CTAB-NCC nanostructured film, g CTAB-NCC/QDs nanocomposite film (Source :Abd Manan et al. [289]).エルゼビアの許可を得て複製
In the food industry, sensors are used to monitor the quality or freshness of food. Moradi et al. [290] developed a pH sensing indicator based on bacterial cellulose nanofibres for monitoring freshness of fish. They used carrot anthocyanins as an indicator. The fabricated sensor displayed wide colour differences from red to gray over the 2–11 pH range. The colour changes were distinguishable whereby deep carmine colour indicates fresh fish, charm pink colour indicates fish is best to eat immediately, and spoiled fish is indicated by jelly bean blue and khaki colours.
Development of Chemical Sensors
Nanocellulose hydrogels structure was previously used to immobilise sulphur and nitrogen co-doped graphene quantum dots as a low-cost sensor for detecting the laccase enzyme [291]. Laccase is the multicopper oxidase family of enzyme. It is involved in the monoelectronic oxidation of several aromatic compounds and aliphatic amines. Therefore, it is usually used in decolouration and coloration products [292, 293]. Therefore, monitoring of laccase activity in commercial products is of great interest. From this study, the authors found the sulphur and nitrogen co-doped graphene quantum dots were self-organised via electrostatic interactions.
Faham et al. [294] described the development of a nanocellulose-based colorimetric assay kit for smartphone sensing of iron, Fe(II) and iron-chelating deferoxamine drug (DFO) in biofluids. They embed curcumin in a transparent bacterial cellulose nanopaper, as a colorimetric assay kit. The assay kit was then used for monitoring of iron and deferoxamine as iron-chelating drug in biological fluid such as urine, blood, saliva and serum. The assay kit can be easily coupled with smartphone technology for colorimetric monitoring of Fe(II) and DFO.
Development of Electrochemical Sensors
Burrs et al. [295] demonstrated the electrochemical biosensor-based glucose for the detection of pathogenic bacteria. The conductive paper used for the development of electrochemical sensor was prepared using graphene-nanocellulose composites. Platinum was electrodeposited on graphene-cellulose composites using pulsed sonoelectrodeposition [296]. Then the sensor was fabricated by functionalising the nanoplatinum with glucose oxidase (GOx ) entrapped in a chitosan hydrogel on the conductive paper. They found that the sensor is extremely efficient for use in electrochemical biosensing with a low detection limit for glucose or pathogenic bacteria.
Ortolani et al. [297] developed an electrochemical sensing device using nanocellulose and single-walled carbon nanohorns (SWCNH) for guanine and adenine determination. Both nanocellulose and SWCNH have large surface area, good conductivity, high porosity and chemical stability. The prepared sensor showed highly sensitive and high electrocatalytic activity towards simultaneous determination of guanine and adenine. The sensor also presented a lower limit detection. Another study conducted by Shalauddin et al. [298] used hybrid nanocellulose/functionalised multi-walled carbon nanotubes (f -MWCNTs for development of electrochemical sensing. The sensor for determination of diclofenac sodium in pharmaceutical drugs and biological fluids samples were used. The presence of –OH groups in the nanocellulose was reported to provide more binding sites for different analytes which ensures an axial modulus rearrangement and incorporation of f -MWCNTs.
Toxicity of Nanomaterial
Although nanomaterial offers many good potential to various industrial sectors, its potential hazard to human and environment could not simply be overlooked [299]. The safety or toxicity issue needs to be addressed by conducting a complete study from various angles. Nanotoxicity or the study of nanomaterials’ toxicity can be classified into several areas namely oxidative stress, genotoxicity, and ecotoxicity. In humans, toxicity can occur through pulmonary, oral and dermal routes. Nanomaterials can affect humans mainly during extraction or production, handling, usage and disposal. Inorganic nanoparticles (NPs) such as ZnO and nickel oxide (NiO) are believed to be more toxic compared to organic nano materials such as nanocellulose. Digested organic NPs are less likely to induce toxicity since the by-products of digestion by stomach acid are simple sugars, although in the case of nanocellulose, the lack of enzyme in human gut capable of degrading cellulose results in the cellulose moving through the gut fairly quickly. A 90-day subchronic toxicity study on Sprague Dawley rats conducted by Ong et al. [300] indicated that no systemic toxicity attributable to 4% dietary consumption of fibrillated cellulose was observed. In the same study, a higher concentration of 30% of nanofibrillated cellulose for 72 weeks also indicated no adverse effects. When present in the air, nanoparticles can also have harmful effects to human health [301]. Multi-walled carbon nanotubes (MWCNT) are possibly one of the most studied NPs for toxicity effects. In the study, Poulsen et al. [302] found that both thick and long as well as thin and short MWCNT induced genotoxic potential and long lasting inflammation and can lead to cardiovascular disease. In contrast, a study on inhalation NCC toxicity in albino rats was conducted and showed no undesirable effects were observed at a maximum concentration of 0.26 mg/L for a period of four (4) hours [303]. At cellular level, cytotoxicity on NCC on different cell types has been studied with most studies concluded that NCC to be non-toxic to a variety of mammalian cells at concentrations below 0.25 mg/L, but can exhibit cytotoxic effects at concentrations of 0.5 mg/L and above [304,305,306,307,308,309]. Primary nanogenotoxicity refers to the possibility of nanoparticles or their by-products to damage DNA directly or indirectly. Secondary genotoxicity is caused by immune system interaction to disturbances cause by nanoparticles. A study by Akerlund et al. [310] indicated that inorganic NPs of Ni and NiO-induced cytokines inflammations which led to secondary genotoxicity in human lung epithelial cells. However, they require further investigation to understand the factors causing this secondary genotoxicity and whether such results are caused by other nanoparticles as well.
NanoTiO2 in wood coatings has been reported to have low toxicity to terrestrial organisms, and no bioaccumulation was observed [311]. Another study conducted on cerium oxide (CeO2 ) nanoparticle or ceria showed that no acute toxicity was observed for Daphnia magna and Thamnocephalus platyurus and D. rerio embryos at test concentrations of 1000, 5000, and 200 mg/L, respectively. However, the same study indicated that a significant chronic toxicity on P. subcapitata was observed with 10% effect concentrations (EC10s) between 2.6 and 5.4 mg/L [312]. Pulido-Reyes et al. [313] explained that the redox cycling between Ce 3+ and Ce 4+ is a unique chemistry of nanoceria which depends on the prevailing environmental conditions. They concluded that in most cerium oxide NP studies, the amount of surface Ce 3+ correlates with toxicity and this can be overcome by blocking the Ce 3+ sites with anions such as phosphates (PO4 3- ) which will reduce reactive oxygen species levels and overcoming oxidative stress. During and after disposal, there is a likelihood that NPs can interact with the environment, whether in soil or aquatic environment. The oxidative stress or carbon-based nanoparticle-induced toxicity is primarily of concern as this condition will affect the oxygen balance in the environment.王ら。 [314] showed that NCCs’ activated oxidative stress in aquatic organisms (Scenedesmus obliquus algae, planktonic crustacean Daphnia magna and freshwater fish larva Danio rerio) at concentrations above 0.1 mg/L. The NCC form, morphology and concentration are believed to be the contributing factors, thus suggesting evaluation of nanocellulose ecotoxicological impacts when used at large scales. One method of reducing the undesired effect to the environment is by providing a mechanism to capture, retain or recycle the NPs during processing. Magnetic NPs (e.g. magnetite) [315] or surface modification can be used for this purpose. Almost all toxicity studies on nanoparticles recommend more research to be conducted on finding suitable analytical techniques and protocols in detecting organic NPs within biological matrices as well as mechanistic and environmental investigations to understand potential NPs risks on the ecosystem [301, 302, 310, 316].
Conclusions
The potential of nanotechnology in wood-based products sector is enormous. Nanotechnology offers the opportunity to change the landscape of wood-based products industry locally and globally. Forest itself via forest plantation can provide sustainable sources towards the creation of new generation of cellulose called nanocellulose that could offer myriads of application in various industrial fields. Besides, nanocellulose is less expensive than carbon-based material (e.g. carbon nanotube, graphene), environmentally friendly and may improve the recyclability and performance of countless products. For instance, nanocellulose can be used to improve the performance of paper and reduce the use of pulp in papermaking, thus resulting in cost saving. Nanocellulose can also be made into nanopaper or thin film for use in paper packaging or other sector such as electronics which offers alternative to non-renewable plastic. Nanocellulose used in wood composite can lead to the development of advanced composites which can be tailored to specific uses. Together with their strength properties and affordability makes them a viable solution to reduce the need for solid wood. Industrial sectors other than wood-based products could also benefit from the use of nanocellulose such as in energy device and sensors. Various nanocellulose-based products for energy storage and energy harvester applications as well as sensor have been developed at laboratory scale. In view of this interesting development, Malaysia has embarked on the production of nanocellulose from various tropical lignocellulosic materials. Unfortunately, the application of nanocellulose at commercial scale in Malaysia is still non-existent. Thus, it requires collective, concert and intensive efforts from various players that include stakeholders, industry and researchers to promote the use of nanocellulose and ensure that nanocellulose could enter the next level of application and commercialisation stage. The use of nanosized material other than nanocellulose has already benefited and also given advantage to wood-based products area which includes pulp and paper, wood composite, wood coating and wood preservative.
データと資料の可用性
All data and materials are available without restriction.
略語
- AgNPs:
-
銀ナノ粒子
- Al2 O 3 :
-
Aluminium oxide
- AuNPs:
-
Gold nanparticles
- BTZ:
-
Benzotriazole
- C:
-
Carbon
- CCA:
-
Chromated copper arsenate
- Cd:
-
Cadmium
- CdS:
-
Cadmium sulphide
- CeO2 :
-
Cerium oxide
- CH2 CHSi(OC2 H 5 ) 3 :
-
Vinyltriethoxysilane
- CMC:
-
Carboxymethyl cellulose
- CNT:
-
カーボンナノチューブ
- CO2 :
-
Carbon dioxide
- CTAB:
-
セチルトリメチルアンモニウムブロミド
- CTAB-NCC:
-
Cetyltrimethylammonium bromide-nanocrystalline cellulose
- CWA:
-
Castor-oil-based waterborne acrylate (CWA)
- DFO:
-
Deferoxamine drug
- FESEM:
-
電界放出型走査電子顕微鏡
- f -MWCNTs:
-
Functionalised multi-walled carbon nanotubes
- FTIR:
-
Fourier transform Infrared
- GOx:
-
グルコースオキシダーゼ
- HALS:
-
Hindered amine light stabilizers
- HNE:
-
Human neutrophil elastase
- ISE:
-
Inorganic solid electrolyte
- LDH:
-
Layered double hydroxide
- LIB:
-
Lithium-ion battery
- LiTFSI:
-
Bis(trifluoromethanesulphonyl)imide lithium salt
- L-NCC:
-
Lignin-coated nanocrystalline cellulose (L-NCC)
- MC:
-
Moisture content
- Mg–Al LDH:
-
Magnesium–aluminium layered double hydroxide
- MPA:
-
3-Mercaptopropionic acid
- MUF:
-
Melamine-urea formaldehyde
- MWCNT:
-
Multi-walled carbon nanotube
- NCC:
-
Nanocrystalline cellulose
- NFC:
-
Nanofibrillated cellulose
- Ni:
-
ニッケル
- NiO:
-
Nickel oxide
- NP:
-
ナノ粒子
- NW:
-
Nanowollastonite
- O:
-
Oxygen
- OH:
-
Hydroxyl
- OLED:
-
有機発光ダイオード
- PA:
-
Polyamide
- PCL:
-
Polycaprolactone
- PCP:
-
Pentachlorophenol
- PDMS:
-
ポリジメチルシロキサン
- PE:
-
Polyethylene
- PEG:
-
Poly(ethylene glycol)
- PEO:
-
ポリエチレンオキシド
- PHB:
-
Poly(3-hydroxybutyrate-co-4-hydroxybutyrate)
- PHRR:
-
Peak heat release rate
- PLA:
-
Polylactic acid
- PMC:
-
Perfluoroalkyl methacrylic copolymer (PMC)
- PMMA:
-
Poly(methyl methacrylate)
- PO4 3- :
-
Phosphates
- POTS:
-
Perfluoroalkyltriethoxysilane
- PP:
-
ポリプロピレン
- PPE:
-
Porcine pancreatic elastase
- PPG:
-
Propylene glycol
- PS:
-
ポリスチレン
- PSU:
-
Polysulphone
- PU:
-
Polyurethane
- PV:
-
Photovoltaic
- QD:
-
量子ドット
- RFID:
-
Radio-frequency identification
- S:
-
Sulphur
- SC:
-
Supercapacitor
- Si NMs:
-
Silicon nanomembranes
- SiO 2 :
-
酸化ケイ素
- SPE:
-
Solid polymer electrolyte
- SPI:
-
Soy protein isolate
- SWCNH:
-
Single-walled carbon nanohorns
- TBOT:
-
Tetrabutyltitanate
- TEM:
-
透過型電子顕微鏡
- TEMPO:
-
2,2,6,6-Tetramethylpiperidin-1-oxyl
- Ti(OC4H9)4:
-
Tetrabutyltitanate
- TiO 2 :
-
二酸化チタン
- TMI-BNT:
-
Transition metal ion-modified bentonite
- TNFC:
-
TEMPO-oxidised nanofibrillated cellulose
- Tyr:
-
Tyrosinase
- UF:
-
Urea formaldehyde
- UP:
-
Unsaturated polyester
- UV:
-
紫外線
- VRFBs:
-
Vanadium redox flow batteries
- VTES:
-
Vinyltriethoxysilane
- WPU:
-
Waterborne polyurethane coating
- WUV:
-
Waterborne
- Zn-Al LDH:
-
Zinc-aluminium layered double hydroxide
- ZnO:
-
酸化亜鉛
- ZnSt2 :
-
Zinc stearate
- ZnSt2 /WUV:
-
Zinc stearate/waterborne UV
ナノマテリアル