金属酸化物ナノ粒子を介した細胞形態機械的摂動の調整
要約
今日、市販製品でのナノ粒子(NP)の使用の増加は、それらの潜在的な有害性の包括的な理解とは一致しません。 NPの物理化学的特性が、細胞内への取り込みと細胞内輸送、運命、および毒性をどのように導くかを検討するには、さらにinvitroでの調査が必要です。これらのナノバイオ相互作用は、特に機械的な観点から、まだ広く取り組まれていません。細胞力学は、細胞の移動、組織の完全性、細胞骨格の再配列を介した分化などのプロセスを調節するため、細胞の健康の重要な指標です。ここでは、SiO 2 によって誘発されるヤング率の変化の観点から、Caco-2およびA549細胞株の弾性摂動をinvitroで調査しました。 NP S およびTiO 2 NP S 。 TiO 2 NPは、SiO 2 と比較して、セルの弾性に対してより強い効果を示しました。 NPは、アクチンネットワークに有意な形態学的および形態計測的変化を引き起こしたため。 TiO 2 NP S Caco-2細胞では弾力性が増加しましたが、A549細胞では反対の効果が観察されました。これらの結果は、細胞の弾力性の変化とNPの毒性との間に相関関係が存在することを示しています。これは、NPの物理化学的特性とテストされた特定の細胞に依存します。
背景
市販製品での人工ナノ粒子(ENP)の大規模な使用は、人間と環境への潜在的な毒性についての認識を高めています[1]。毒性の分子メカニズムに光を当てることを目的として、これまで多くのinvitroおよびinvivo調査が実施されてきました[2、3]。しかし、ナノ粒子(NP)と生物の間の相互作用を理解することは、標準化された操作手順がないためにかなり困難であり、現在の物議を醸す文献データが利用可能になりました[4、5]。 NPの悪影響は、その物理化学的特性と、テストされた特定の細胞または生物に厳密に依存することが確立されています[6]。このため、NPの特性評価は、信頼できるデータを実現するための基本です[7]。金属酸化物NPは、主に市販製品に広く普及しています[8]。これらの中で、アモルファスSiO 2 NPと結晶性TiO 2 NPは、医薬品や化粧品への添加物として、またヘルスケア製品、プリンタートナー、塗料、食品包装、食品添加物として、幅広い産業分野で使用されています[9、10]。したがって、これらのNPは、さまざまな経路(摂取、吸入、皮膚浸透)を介して生物にアクセスできる可能性があります[11]。例としては、TiO 2 をベースにした食品がありますが、これに限定されません。 NP(商用ラベルでE171とラベル付けされています)およびSiO 2 NP(商用ラベルのE551、E554、E556)は、大幅に成長しています[12、13、14]。 SiO 2 に関する現在の研究 NPとTiO 2 NPは、重要な細胞メカニズムに積極的に干渉することを示唆しています。たとえば、サイトカイン放出を刺激し(したがって炎症を促進し)[15,16,17]、腸の微絨毛を損傷し[18、19]、ROS産生を誘発し[20]、ATP合成を阻害し[21]、誘発することが証明されています。遺伝子毒性[22、23、24、25、26]。しかし、これらのNPが細胞力学と相互作用するかどうかを調査した研究はほとんどありません[27]。このトピックについてはさらに調査が必要です。細胞接着と細胞骨格の再配列は、細胞の恒常性を実際に維持するために重要です[28]。細胞骨格構造の変化は、細胞力学を混乱させ、細胞の弾力性と移動のダイナミクスに影響を与える可能性があります[29]。この研究では、20nmのSiO 2 の生体力学的効果を注意深く評価しました。 NPとTiO 2 Caco-2およびA549細胞上のNP。これは、NPに曝露された組織に似た最良のモデルです。我々は予備的にそれらの侵入メカニズムを調査し、細胞生存率、膜損傷、およびスーパーオキシドジスムターゼ(SOD)とマロンジアルデヒド(MDA)の活性化とともにROS産生を評価しました。次に、原子間力顕微鏡(AFM)によるNPインキュベーション時の細胞弾性(ヤング率)の変化を特徴づけることに焦点を当てました。私たちの結果は、ヤング率の変化によって確認されるように、NPが皮質アクチンの有意な再編成を誘発する可能性があることを示しています。特に、SiO 2 の主要な生体適合性 TiO 2 の慢性毒性に対するNP NPが観察されています。細胞毒性調査と生体力学的特性を組み合わせるという私たちのアプローチは、NP毒性評価のプロトコルを標準化するための新しい潜在的な方法を表しています。
メソッド
アモルファスSiOの合成 2 NP
三元W / Oマイクロエマルジョンは、水、有機溶媒(シクロヘキサン、J.T。ベイカー)、界面活性剤(トリトンX-100、シグマアルドリッチ)をマルビンディらの方法に従って混合することにより、室温で調製された。 [25]。簡単に説明すると、880μLのTriton X-100、3.75 mLのシクロヘキサン、170 mLの水、および50μLのTEOS(98%、Sigma-Aldrich)を混合し、30分間撹拌しました。その後、30μLのNH 4 OH(28.0–30.0%、Sigma-Aldrich)をマイクロエマルジョンに添加しました。 24時間後、懸濁液を遠心分離(4500 rpm)、続いてエタノール(98%、Sigma-Aldrich)、およびmilliQ水で5回洗浄することにより分離しました。次に、ナノ粒子を水に分散させました。
TiO 2 の合成 NP
TiO 2 NPは、Leena etal。によって記述されたゾルゲル法に従って調製されました。 [30]いくつかの変更があります。簡単に説明すると、チタン(IV)イソプロポキシド(TTIP; 99.9%Sigma-Aldrich)を、酸性条件(pH 3)で攪拌しながらエタノールとmilliQ水(5:1:1)の溶液に滴下しました。 NPを最初に30°Cで5時間、次に430°Cで3時間インキュベートして、白色のナノ粉末を得ました。
TEMの特性評価
透過型電子顕微鏡(TEM)の特性評価は、加速電圧100 Kv(JEOL USA、Inc。)で動作するJEOL Jem1011顕微鏡を使用して実行されました。 TEMサンプルは、カーボンコーティングされた銅グリッド(Formvar / Carbon 300 Mesh Cu)にNPの希薄溶液を水中に滴下することによって準備されました。
DLSおよびζ電位測定
SiO 2 の平均流体力学的サイズとゼータ電位 NPとTiO 2 NPは、動的光散乱(DLS)と、633nmで動作する4.0 mWHeNeレーザーとアバランシェフォトダイオード検出器(モデルZEN3600、Malvern Instruments Ltd.、Malvern、英国)。測定は、水溶液中、およびFBS(Sigma-Aldrich)を添加した細胞培養培地(DMEM、高グルコース、Sigma-Aldrich)、10%および20%pHで25°Cで行いました7)。 DLS測定値とゼータ電位の平均値を取得するために、2つの独立した技術的複製を使用して各サンプルを3回実行しました。
XRDの特性評価
TiO 2 の結晶相分析用の粉末X線回折(XRD) NPは、フィルター処理されたCu-Ka放射線を使用して、Bragg-Brentano反射ジオメトリのRigaku回折計で実行されました。 XRDパターンは、0.02の2Q増分と、2秒/ステップの固定カウント時間を使用して、ステップスキャンによって2Q¼20–80の範囲で記録されました。
細胞培養
Caco-2(ATCC®HTB-37™)およびA549(ATCC®CCL-185™)は、100 U / mLペニシリンおよび100mg / mLストレプトマイシンを添加した50μMグルタミンを含むDMEMで維持されました。 FBSの割合はA549で10%、Caco-2細胞で20%でした。細胞は、空気/ CO 2 の比率が95〜5%の加湿制御雰囲気で培養されました。 、37°Cで。
SiOの細胞内取り込みの測定 2 NPSとTiO 2 NP
10 5 Caco-2およびA549細胞を6ウェルプレートの1mLの培地に播種しました。 37°Cで24時間培養した後、培地をSiO 2 を含む新しい培地と交換しました。 NPとTiO 2 15μg/ mlおよび45μg/ mlの濃度のNP。 37°Cで48時間、72時間、96時間培養した後、DMEMを除去し、細胞をPBS(pH 7.4)で4回洗浄して、細胞膜に結合している可能性のあるNPを除去しました。細胞をトリプシン処理し、自動細胞計数チャンバーを使用して計数した。 36万個の細胞を200μLのmilliQに懸濁し、HCl / HNO 3 で処理しました。 3:1( v / v )および5 mLに希釈:得られた溶液を分析して、SiおよびTi含有量を評価しました。元素分析は、Varian Vista AX分光計を使用した誘導結合プラズマ原子発光分析(ICP-AES)によって実行されました。
WST-8アッセイ
Caco-2およびA549細胞を96ウェルマイクロプレートに5×10 3 の濃度で播種しました。 安定化の24時間後の細胞/ウェル。 NPストック溶液(SiO 2 NPとTiO 2 NP)を15μg/ mlおよび45μg/ mlで細胞培地に添加しました。細胞を24時間、48時間、72時間、および96時間インキュベートしました。エンドポイントで、標準的なWST-8アッセイ(Sigma-Aldrich)を使用して細胞生存率を測定しました。アッセイは、De Matteis etal。に以前に記載された手順に従って実施された。 [31]。データは平均±SDとして表されました。
LDHアッセイ
Caco-2およびA549細胞をSiO 2 で処理しました NPとTiO 2 WST-8アッセイで報告された手順に従ったNP。乳酸デヒドロゲナーゼ(LDH)アッセイは、CytoTox-ONEホモジニアスメンブレンインテグリティアッセイ試薬(Promega)を製造元の指示に従って適用することにより、マイクロプレート上で実行されました。培地を回収し、Bio-Radマイクロプレート分光光度計を使用して490nmでの吸光度を読み取ることによりLDHのレベルを測定しました。データは平均±SDとして表されました。
DCF-DAアッセイ
Caco-2およびA549細胞を96ウェルマイクロプレートに播種し、SiO 2 で処理しました。 NPとTiO 2 15μg/ mlおよび45μg/ mlの最終濃度のNP。細胞とNPの相互作用の24時間、48時間、72時間、および96時間後、De Matteis et al。によって報告された手順に従って、DCF-DA(Sigma)アッセイをマイクロプレート上で実行しました。 [32]データは平均±SDとして表されました。
SODアッセイ
Caco-2およびA549(15μg/ ml、45μg/ mlで24時間、48時間、72時間、および96時間インキュベート)細胞抽出物は、[33]に記載されているプロトコルに従って調製しました。アッセイは、3種類すべてのSOD(Cu / ZnSOD、MnSOD、およびFeSOD)を測定するSODアッセイ(Cayman Chemical Company、Michigan、OH、USA)を適用することにより、マイクロプレート上で実行されました。このアッセイでは、キサンチンオキシダーゼとヒポキサンチンによって生成されるスーパーオキシドラジカルの検出にテトラゾリウム塩を使用しました。 SODの1単位は、スーパーオキシドラジカルの50%の不均化を示すために必要な酵素の量として定義されます。 SOD活性は、Bio-Radマイクロプレート分光光度計を使用して440〜460nmの吸光度を読み取ることで測定しました。
MDAアッセイ
Caco-2およびA549(15μg/ ml、45μg/ mlで24時間、48時間、72時間、および96時間インキュベート)細胞抽出物は、前述の手順[33]に従って調製しました。脂質過酸化(MDA)アッセイキット(Abcam)を適用することにより、マイクロプレート上でアッセイを実施しました。サンプル中のMDAをチオバルビツール酸(TBA)と反応させて、MDA-TBA付加物を生成しました。このルートでは、MDA-TBA付加物の形成中に生成される赤色の分光光度測定を行いました。これは、Bio-Radマイクロプレート分光光度計を使用して532 nmでの吸光度を読み取ることにより、(nmol / mgタンパク質で)定量化できます。
>CLSM分析
細胞を10 5 の濃度で24ウェルプレートに播種しました 細胞/ウェルおよび連続的にSiO 2 とインキュベート NPとTiO 2 15μg/ mlおよび45μg/ mlの濃度で24時間、48時間、72時間、および96時間のNP。処理後、各時点で、ナノ粒子を含む培地を除去し、細胞を0.25%グルタルアルデヒド( v )で固定したPBSで3回洗浄しました。 / v PBS、Sigma-Aldrich)で20分間、最後に0.1%Triton( v )で透過処理します。 / v PBS、Sigma-Aldrich)で5分間アクチン染色には、ファロイジン-ATTO 488(Sigma-Aldrich)を1μg/ mlの濃度で30分間使用しました。核は、1μg/ mlの濃度で7分間DAPI(Sigma-Aldrich)を使用してマークされました。レーザー走査型共焦点顕微鏡は、Axio Observer Z1(Zeiss)倒立顕微鏡を備えたZeiss LSM700(Zeiss)共焦点顕微鏡で、イメージングに×100、1.46数値開口油浸レンズを使用して実施しました。共焦点データファイルはZEN2010ソフトウェア(Zeiss)を使用して処理され、形態計測定量化(F-アクチンのコヒーレンシーと統合密度)はImageJ1.47分析ソフトウェアを使用して15個のセルで実行されました。 OrientationJプラグインは、ローカル近傍の構造テンソルの測定に基づいて、共焦点取得でROIの特定のシーケンスを選択することにより、コヒーレンシパラメーターを定量化するために使用されました。同時に、ソフトウェアは、アクチン繊維が配向した程度を表す配向とコヒーレンシーの値を計算しました。より無秩序な繊維は0に近い値を持ちますが、完全に整列した繊維は約1のコヒーレンシ値を示します[34]。統合密度は、細胞内のアクチン繊維の量を定量化するために、共焦点取得のROIのピクセル値の合計によっても計算されました。
AFM分析
Caco-2およびA549細胞は、10 5 の濃度でプラスチックシャーレ(コーニング)に播種されました。 細胞/ウェルで、コンフルエンスの70〜80%まで増殖します。次に、細胞を45μg/ mlのTiO 2 で処理しました。 NP S およびSiO 2 72時間のDMEMのNP。続いて、NPを除去し、細胞をPBSで洗浄した。グルタルアルデヒド0.25%を使用して細胞を20分間固定した後、PBSで洗浄しました。測定は、倒立光学顕微鏡(Zeiss Observer Z1、Zeiss GERMANY)に取り付けられた高度な走査型プローブ顕微鏡(Bioscope Catalyst、Bruker Inc.、USA)によって実施されました。システム全体は、環境の機械的振動に対して絶縁体として機能するベース上に配置されます。 AFM実験は、V字型のBrukerのSharp Microlever(MSNL、チップC)を使用して、力-体積モードで実行されました。これは、公称ばね定数が0.01 N / mの高感度窒化ケイ素カンチレバーです。この値は、AFM取得を実行する前に、サーマルチューン法[35]によって正確に推定されました。使用したパラメータは次のとおりです。スキャン領域50μm、ランプレート3 Hz、FVスキャンレート0.03 Hz、トリガーしきい値100 nm、サンプル数128、ライン64あたりのサンプル、およびライン64。ヤング率(E)は次のように決定されました。 20個の細胞から、核領域に対応する25個の力-距離曲線と細胞質領域の25個の曲線が抽出されました。抽出された曲線から導出されたアプローチデータ(接触点から最大力値まで)セットは、修正されたSneddonモデルに適合しました:
$$-{k} _ {\ mathrm {c}} {\ delta} _ {\ mathrm {c}} =\ frac {2 Etg \ alpha} {\ pi \ left(1-{\ nu} ^ 2 \右)} {\ left(z-{\ delta} _ {\ mathrm {c}} \ right)} ^ 2 $$ここで z およびδ c 実験的な荷重データ(それぞれ高さとカンチレバーのたわみ)、α 先端の半角、 k c はカンチレバーの弾性定数値であり、ν はポアソン比です(生物学的サンプルの場合は0.5と想定)。適合アルゴリズムでは、接触点は適合変数として扱われ、接着力が考慮され、20個のセルで取得されました。
統計分析
データは、平均値および関連する標準偏差として表されました。異なる平均値間の差は、Student t の実行で統計的に有意であると見なされました。 p でテストする 値˂0.05(<0.05 *、<0.01 **、および<0.005 ***)。
結果
SiO 2の特性評価 NPとTiO 2 NP
SiO 2 NPとTiO 2 NPは、サイズ分布が狭く制御されたNPを取得するために、さまざまな再現性のある合成ルートで合成されています(「方法」のセクションを参照)。次に、NPは、TEM、DLS、ζ電位、およびXRDによって、水中およびタンパク質源(FBS)の濃度が異なる細胞培養培地(DMEM)の両方で深く特徴付けられました。メディアタンパク質はNPの表面を覆い、物理化学的特性を変化させ、生物学的効果を変化させる可能性があるため、これは非常に重要です[36]。 TEM分析は、SiO 2 NPは球形で、平均直径は20±2 nmです(図1a)。 TiO 2 NPのサイズは似ていますが(25±5 nm)、形態が異なります(図1)。 96 hの水中で実施されたDLS測定により、SiO 2 の流体力学的半径が21±7nmおよび27±12nmであることが確認されました。 NPとTiO 2 それぞれNP(図1bおよび図1e)。予想通り、これらのデータはTEM観察とよく一致しています。 ζ電位分析では、SiO 2 の水中の表面電荷値が-45±3mVであることも確認されました。 NPおよびTiO 2 の場合は-50±3mV NP(図1c、f)。予想通り、NPの物理化学的特性は細胞培養培地に接種すると変化しました。 DLSは、特に20%のFBSを添加したDMEMの存在下で、NPサイズの大幅な増加を確認しました(表1)。特に、SiO 2 NPは29±9nmのサイズを示しましたが、TiO 2 NPは、96時間後に41±14nmまで増加しました。 DMEM測定(FBSありまたはなし)で観察されたDLSピークの拡大は、NPの凝集の兆候であり、培地のイオン強度によって促進される可能性があります(データは示していません)。また、ゼータ電位測定は、両方のNPの表面電荷がより負の値にシフトしたことを示しました。 この大きな時間依存現象は、NPの表面に吸着された細胞培養培地中の血清タンパク質の存在によって誘発された非常に安定したタンパク質コロナ形成[37、38]によるものでした:NPのサイズと電荷は関数として変化しますFBS濃度の。
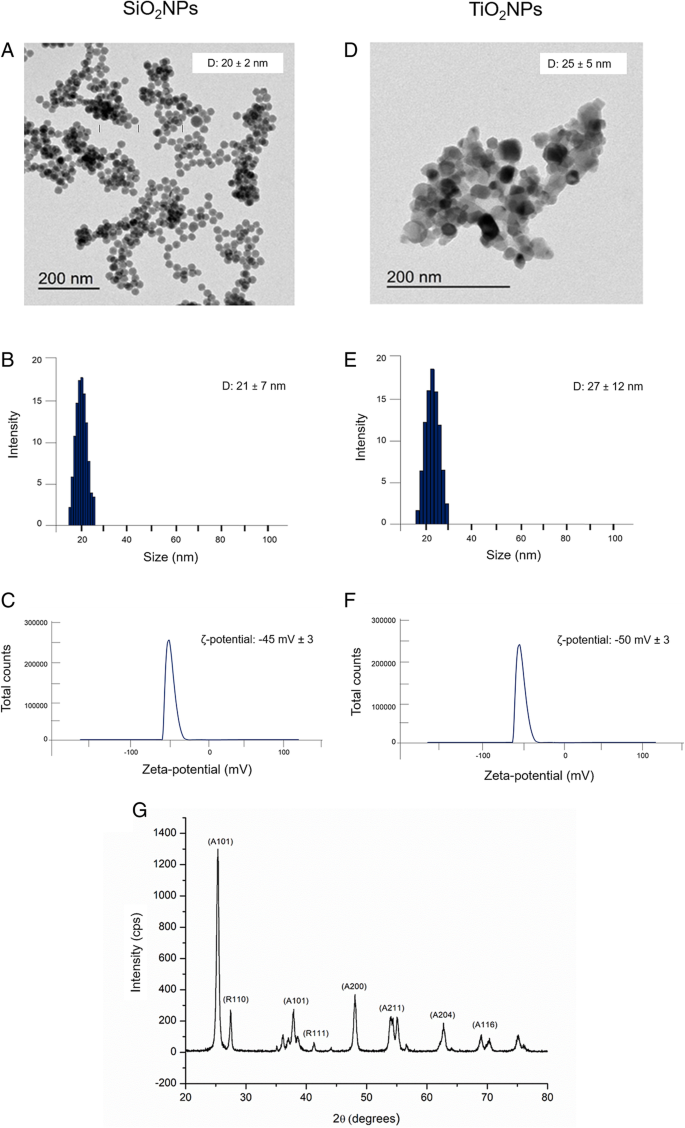
SiO 2 の特性評価 NPとTiO 2 水中のNP。 a – d 代表的なTEM画像。 b – e 動的光散乱(DLS)および c – f ζ電位測定。 g TiO 2 のX線回折分析(XRD)パターン NP
TiO 2 のXRDパターン NP S 、430°Cで煆焼され、アナターゼとルチル結晶相の混合物を示しました(図1g ) 。 2θ=25.4°(101)、48.1°(200)、54.1°(211)、62.4°(204)、および68.8°(116)の主要なピークは、標準のJCPDSデータ(カード番号:21–1272)。ルチル相は、27.5°(110)、36.2°(101)、および41.2°(111)に回折ピークで表されました。
Caco-2およびA549細胞におけるNPの取り込み
SiO 2 の量を定量化するため NPとTiO 2 NP S 細胞に取り込まれた予備調査として、溶解した細胞に対してICP-AES元素分析を行いました。細胞を15μg/ mlおよび45μg/ mlのNPで処理しました。実験データにより、SiO 2 の存在が確認されました。 NPとTiO 2 時間依存の内在化効率を持つ両方の細胞株のNP(図2a)。 TiO 2 NPは、SiO 2 に関してより大きな取り込みを示しました。 NP。これはCaco-2で特に顕著であり、Ti含有量は72時間後と96時間後にそれぞれ8.2±0.4μgと9.7±0.031μgの細胞内濃度に達しました。 A549で検出されたTiの量は、72時間後に5±0.599μg、96時間のインキュベーション時間後に7.12±0.11μgであることがわかったため、より低くなりました。 SiO 2 NPは、TiO 2 と比較して細胞による取り込みが少なかった Caco-2で内部移行がより顕著であったとしても、NP。この場合も、実際には、内部移行したSiO 2 の量 Caco-2細胞のNPは、72時間後は4.69±0.031μg、96時間後は5.78±0.045μgでした。値はA549で減少し、72時間後に2.58±0.045μg、96時間後に4.7±0.04μgを定量化しました。
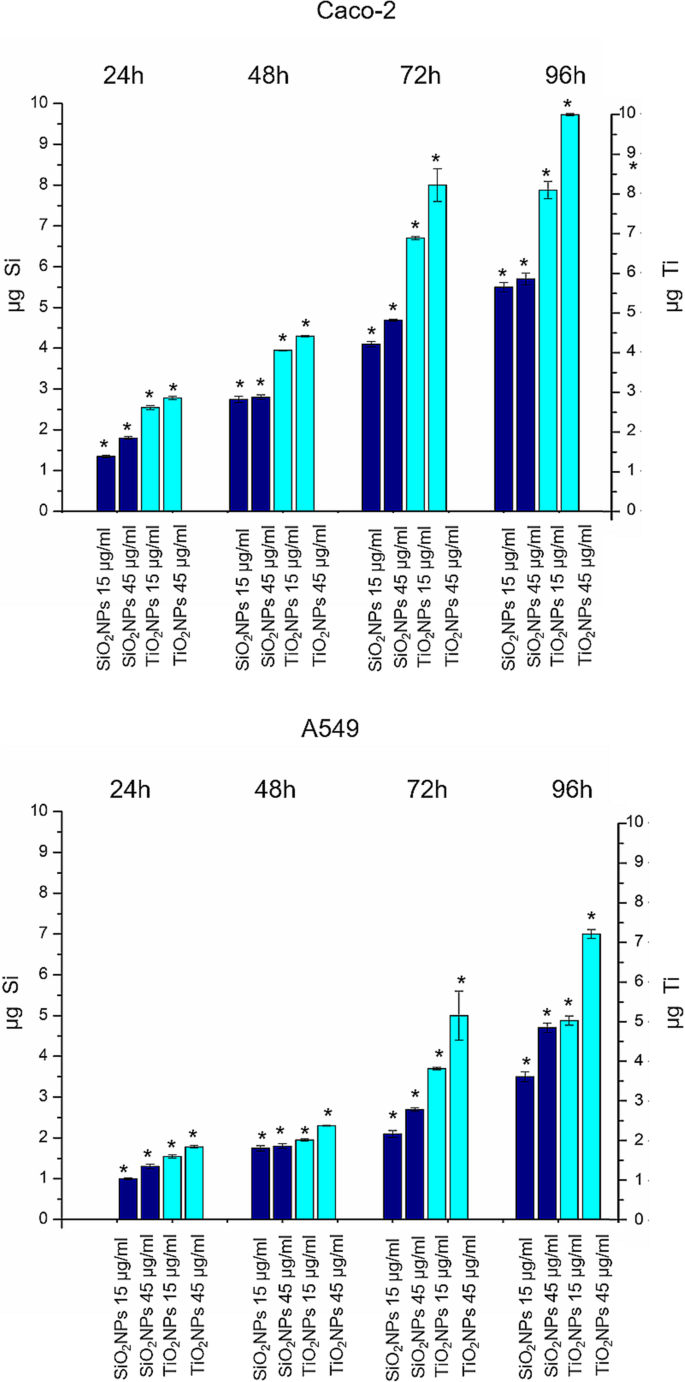
TiO 2 NPとSiO 2 15μg/ mlおよび45μg/ mlのTiO 2 に曝露されたCaco-2およびA549細胞株におけるNPの蓄積 NPとSiO 2 24 h、48 h、72 h、および96hのNP。次に、細胞を回収し、生細胞をカウントし、360,000個の細胞(μgTiおよびμgSi)でTiおよびSi含有量を測定しました。 3つの独立した実験からの平均±SDとして報告されたデータ。 p の曝露細胞と対照細胞の統計的有意性 値<0.05(<0.05 *、<0.01 **、および<0.005 ***)
CaCo-2およびA549に対するNPの影響:細胞生存率、膜損傷、およびROS産生
Caco-2およびA549細胞の生存率は、WST-8アッセイで評価されました。 SiO 2 による処理 NPとTiO 2 NPは、テストした両方の細胞株で、用量依存的な生存率のわずかな低下を引き起こしました(図3)。 TiO 2 NPは、SiO 2 に関して細胞毒性の増強を誘導しました NP、およびCaCo-2細胞の細胞生存率は、TiO 2 で処理すると、A549よりも影響を受けました。 NP。特に、45μg/ mlのTiO 2 で処理したCaco-2では、生存率が約40%低下することが観察されました。 72時間のNP。この減少は、96時間後にさらに50%まで低下しましたが、A549細胞株では、TiO 2 NPは、96時間の処理後にのみ生存率の30%の低下を誘発しました。 LDH放出とROS生成は、TiO 2 への曝露時にCaco-2およびA549細胞で評価されました。 NPとSiO 2 NP。図4a、bに示すように、NPは、生存率の結果と密接に一致して、細胞膜のポレーション(および実際にLDHの放出)を誘導しました。この効果は、特にTiO 2 で、A549に関してCaco-2でより明白でした。 最高の時点(72および96 h)でのNP治療。 LDH放出率は、96時間の曝露後、未処理(コントロール)細胞と比較して約160%の増加に達しました。 ROSの生成は、NPによって誘発される主要な影響の1つであるため、広く研究されてきました[39]。この現象は生物学的抗酸化防御反応を妨害します[40]が、実際の作用機序はまだ調査中であることに言及することが重要です。潜在的なNP誘発酸化ストレスはDCFH-DAアッセイによって推定されました。予想通り、NPと細胞間の相互作用は、用量依存的にROSの生成を刺激し、TiO 2 に対するCaco-2の強い効果を示しました。 NP治療(図4c、d)。試験した最高濃度で、放出の割合は対照細胞に対して165%の値に達しました。
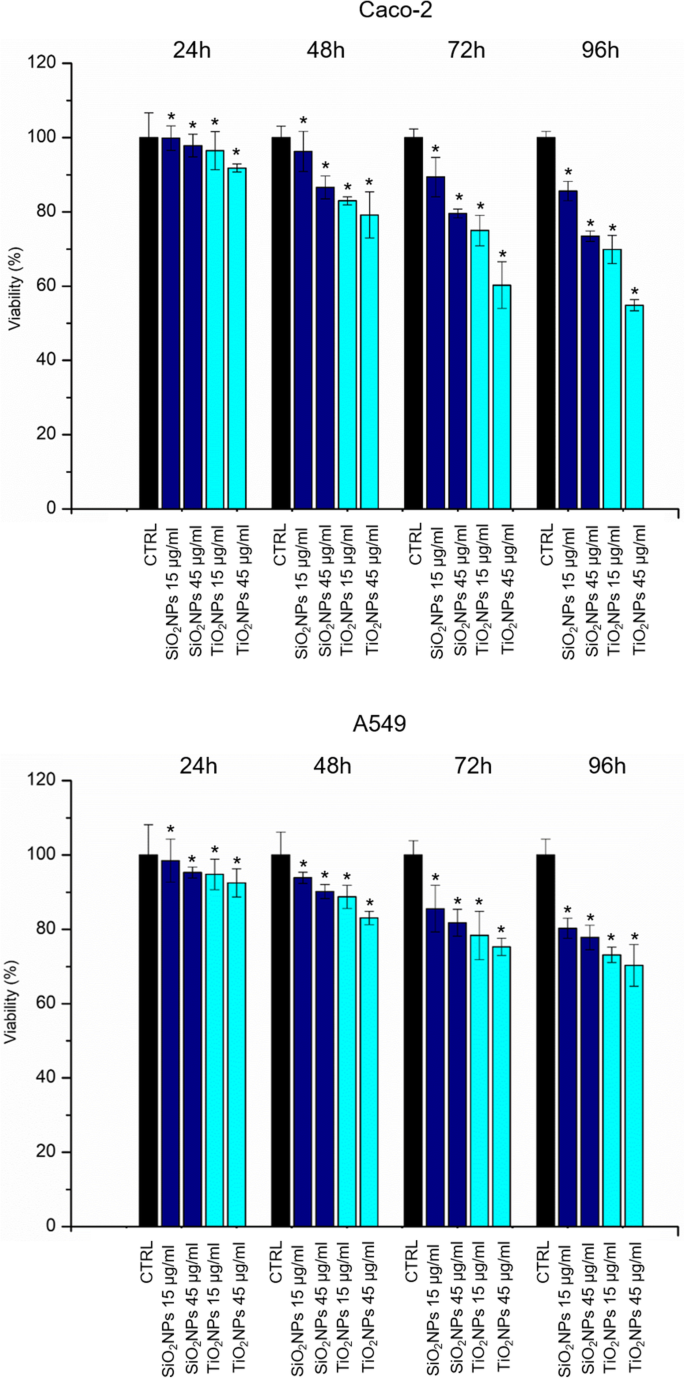
Caco-2の生存率アッセイ(WST-8)( a )およびA549( b )TiO 2 の2つの用量(15μg/ mlおよび45μg/ ml)への24時間、48時間、72時間、および96時間の曝露後の細胞 NPとSiO 2 NP。 NP処理細胞の生存率は、未処理のコントロール細胞に対して正規化されました。ポジティブコントロール(P)として、細胞を5%DMSOとともにインキュベートしました(データは示していません)。 3つの独立した実験からの平均±SDとして報告されたデータは、対照と比較して統計的に有意であると見なされます( n =8) p の場合 値<0.05(<0.05 *、<0.01 **、および<0.005 ***)
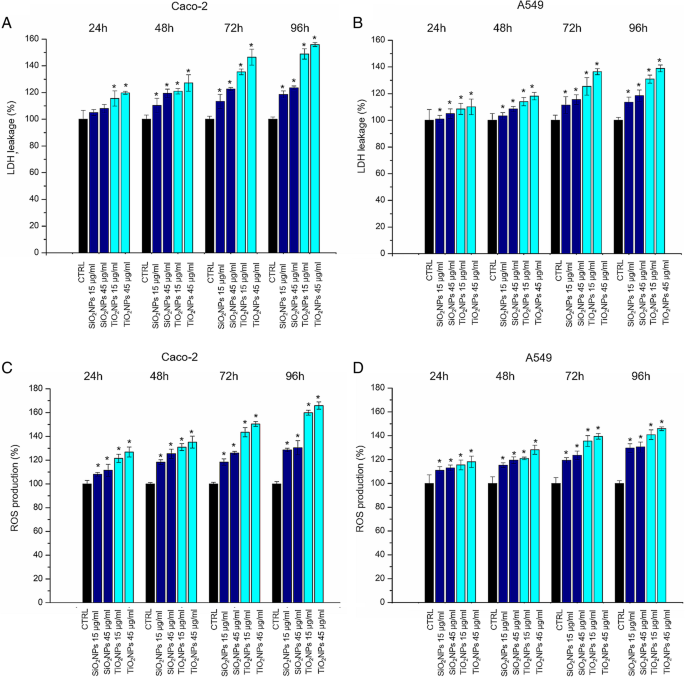
LDH( a – b )およびROS( c – d )Caco-2およびA549細胞でのアッセイ。細胞を15μg/ mlおよび45μg/ mlのTiO 2 とともにインキュベートしました。 NPとSiO 2 24 h、48 h、72 h、および96hのNP。ナノ粒子で処理された細胞のLDH漏出の割合は、未処理の対照細胞と比較して表されます。陽性対照(P)は、0.9%TritonX-100による細胞の処理で構成されていました。 500%のLDH増加(データは示していません)。 15μg/ mlおよび45μg/ mlのTiO 2 でCaco-2およびA549細胞を曝露してROSレベルを記録しました。 NPとSiO 2 100μMDCFH-DAとインキュベートした24時間、48時間、72時間、および96時間のNP。細胞の蛍光を測定した。ポジティブコントロール(P)として、細胞を500μMH 2 とインキュベートしました。 O 2 約を示しています。 DCFH-DAが300%増加します(表示されていません)。 3つの独立した実験からの平均±SDとして報告されたデータは、対照と比較して統計的に有意であると見なされます( n =8) p の場合 値<0.05(<0.05 *、<0.01 **、および<.005 ***)
Caco-2およびA549細胞の抗酸化活性と脂質過酸化に対するNPによって誘発される効果
SOD酵素は酸化ストレス誘導後の抗酸化防御システムに関与しています。この酵素は、反応性の高いスーパーオキシド(O 2 •− )過酸化物への陰イオンH 2 O 2 [41]。 SiO 2 とのインキュベーション後、Caco-2とA549の両方でSOD酵素活性の用量依存的な低下が観察されました。 NPとTiO 2 さまざまな時点(24〜96 h)でのNP(15μg/ ml、45μg/ ml)(図5a、b)。細胞毒性評価と密接に一致して、効果はTiO 2 に対するCaco-2でより明白でした。 NP曝露。たとえば、SOD活性レベルは、コントロールの4.1±0.2 U / mlから45μg/ mlのTiO 2 に曝露されたCaco-2細胞の1.03±0.325U / mlに減少しました。 NP、96時間後。同じ濃度のSiO 2 への暴露 NPはSOD活性を1.45±0.12U / mlに減少させました。 MDAベースのアッセイを使用して、潜在的なROSを介した脂質過酸化をチェックしました。これは、細胞の酸化ストレスをチェックする別の方法です。 [42] MDAの細胞レベルは、Caco-2とA549の両方の2種類のNPに曝露した後に増加しました(図5c、d)。予想通り、増加したMDAレベルは濃度と曝露時間に比例していました。
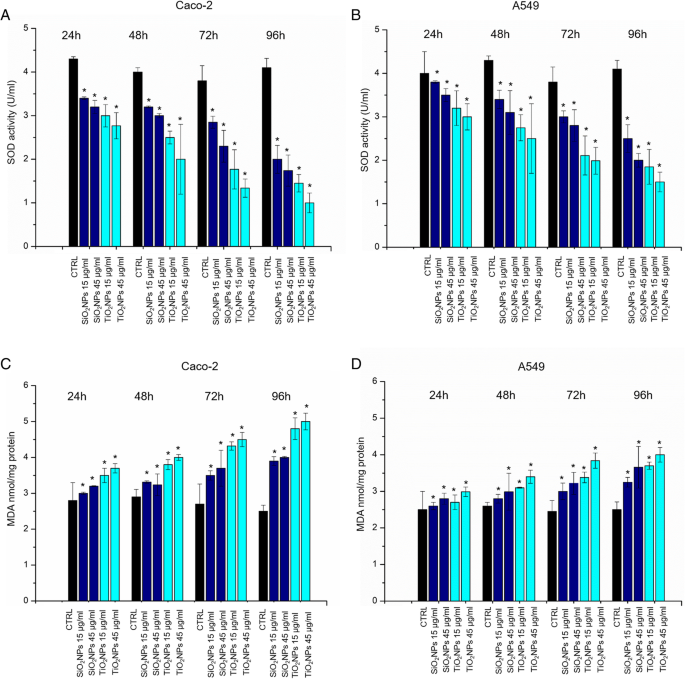
a – d Caco-2およびA549細胞でのSODおよびMDAアッセイ。細胞を15μg/ mlおよび45μg/ mlのTiO 2 とともにインキュベートしました。 NPとSiO 2 24 h、48 h、72 h、96hのNP。 SODアッセイでは、キサンチンオキシダーゼとヒポキサンチンによって生成されるスーパーオキシドラジカルの検出にテトラゾリウム塩を使用しました。標準曲線をポジティブコントロールとして使用しました(データは示していません)。 MDAレベルは、MDA-TBA付加物(OD =532 nm)の定量化によって検出されました。 3つの独立した実験からの平均±SDとして報告されたデータは、対照と比較して統計的に有意であると見なされます( n =8) p の場合 値<0.05(<0.05 *、<0.01 **、および<0.005 ***)
NPによって誘発される機械的効果
15μg/ mlおよび45μg/ mlのSiO 2 とインキュベートしたCaco-2およびA549の共焦点顕微鏡分析 NPとTiO 2 24 h、48 h、72 h、および96hのNPが図1および2に報告されています。対照のCaco-2細胞は、密着結合と刷子縁が頂端側にある腸細胞に似た形態を示した[43]。 NPで処理すると、細胞の密着結合が崩壊し、細胞のパターンが分離され、細長い形状になりました。これらの効果は、細胞をTiO 2 で処理した場合により顕著になりました。 45μg/ mlで72時間のインキュベーション時間のNP。アクチンパターンの関連する変化と細胞形態の変化を示しています。未処理のA549細胞は小石のような形状と機能的な細胞間接着を示しましたが[44]、NPによる処理は細胞間接触を減少させ、細胞形態をより細長い形状に変更しました。図8は、拡大された共焦点図を示しています。これにより、アクチン分布の変化を検出できます。 NP曝露後のアクチンネットワークの組織の変化(45μg/ mlのSiO 2 の72h NPとTiO 2 NP)は、ImageJソフトウェアを使用して蛍光密度とコヒーレンシーによって定量的に分析されました(図9)。統合された密度によってアクチンの量を定量化でき、コヒーレンシーによって周囲と比較した繊維配向の程度に関する情報が得られたため、これら2つのパラメーターを特に選択しました[45]。未処理のCaco-2細胞の密度値は129.4±16であり、これはNP処理の影響を受けませんでした。 SiO 2 に曝露した後の値は、127.7±20および128.5±18でした。 NPとTiO 2 それぞれNP、図9a)。同様に、アクチン染色ネットワークの密度も、処理の前後でA549で同じままでした(ネガティブコントロール、SiO 2 の場合は68.4±14、67.9±15、および67.7±18 NP、およびTiO 2 それぞれNP、図9b)。 NP処理はアクチンの量の変化を誘発しませんでしたが、コヒーレンシ分析は異なるアクチンの空間的再編成を示唆しました。 Caco-2では、SiO 2 の処理済みセルのコヒーレンシ値 NP(0.16±0.04)およびTiO 2 の場合 NP(0.09±0.02)処理は、コントロール(0.26±0.03)の処理と比較して減少しました(図9c)。 A549細胞でさえ、SiO 2 と相互作用した後、コヒーレンシーが低下しました。 NPとTiO 2 コントロールセル(0.4±0.03)と比較したNP(0.2±0.07および0.158±0.04)(図9d)。したがって、NPはアクチンネットワークの有意な再編成を誘発し、アクチンの等方性配向を示しましたが、発現したアクチンの総量は変化しませんでした。細胞骨格の再配列に加えて、核/細胞質比によって記述される領域も分析しました。 N / C比の値は、核領域と全細胞領域の比率として計算されました(15個のセルで測定)。 We observed opposite values following the treatment with 45 μg/ml of NPs for 72 h with significant statistical validity. In particular, the ratio was (0.40 ± 1.7) in untreated Caco-2 cells, and this increased up to 0.554 ± 0.09 and 0.62 ± 0.12 after SiO2 NPおよびTiO 2 NP exposure (Fig. 9e). The trend was different in A549. The nuclear/cytoplasm ratio dropped down upon exposure to NPs from values of 0.550 ± 0.04 for control cells to 0.334 ± 0.06 for SiO2 NPs and 0.225 ± 0.09 for TiO2 NP。 After the morphological investigations, we analyzed the elastic properties of cells after exposing them to 45 μg/ml of SiO2 NPとTiO 2 NPs for 72 h by AFM, in force–volume mode. We measured the different elasticity expressed by Young’s modulus values in the nuclear and cytoplasmic region. Caco-2 cells displayed Young’s modulus value of 105 ± 25 kPa for nuclear region and 47 ± 21 kPa for the cytoplasm. After SiO2 NP treatment, we observed a reduction of value to 42 ± 8 kPa for the nucleus and 19.59 ± 2 kPa for the cytoplasm. The effects were more evident after treatment with TiO2 NPs:the Young modulus for the nucleus was 27 ± 4 kPa and 18 ± 4 kPa for the cytoplasm (Fig. 10a). We found an opposite outcome concerning the elastic properties of A549 cells. In this case, Young’s modulus was 129 ± 24 kPa for the nuclear region and 147 ± 26 kPa for the cytoplasmic area. After SiO2 NP treatment, the values of elasticity increased to 152 ± 23 kPa for nucleus and 152 ± 25 kPa for cytoplasm. When cells were doped with TiO2 NPs, Young’s modulus values drastically increased to 372 ± 60 kPa for nucleus region and 549 ± 40 kPa for cytoplasmic region (Fig. 10b).
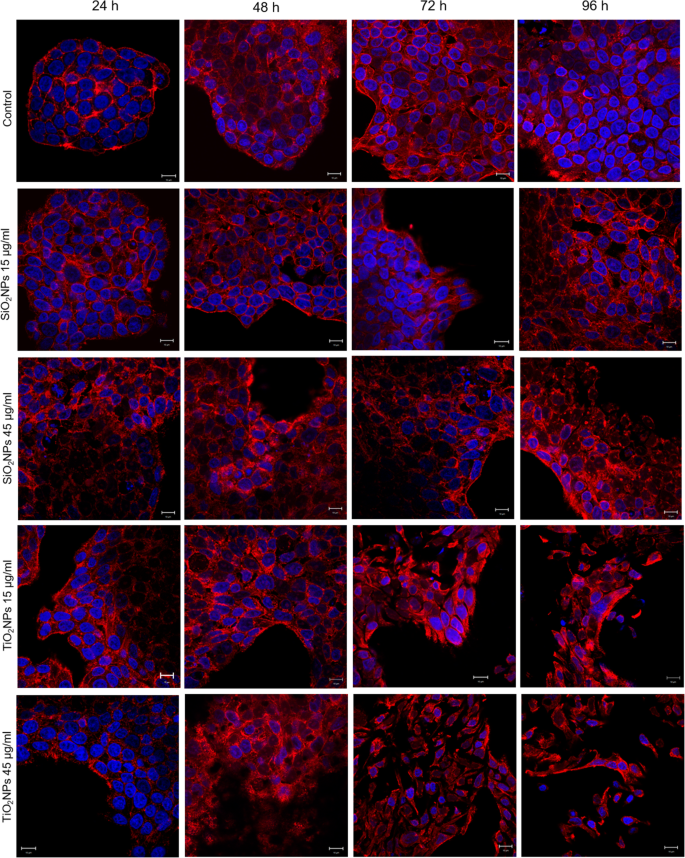
Effects of SiO2 NPS and TiO2 NPs on actin network of Caco-2 cells. Caco2 were treated with 15 μg/ml and 45 μg/ml of NPs for 24 h, 48 h, 72 h, and 96 h; cells were fixed and then stained with Phalloidin–ATTO 488 and DAPI. The 2D images of cortical actin were acquired by a Zeiss LSM700 (Zeiss) confocal microscope equipped with an Axio Observer Z1 (Zeiss) inverted microscope using a × 100, 1.46 numerical aperture oil immersion lens. All data were processed by ZEN software (Zeiss)
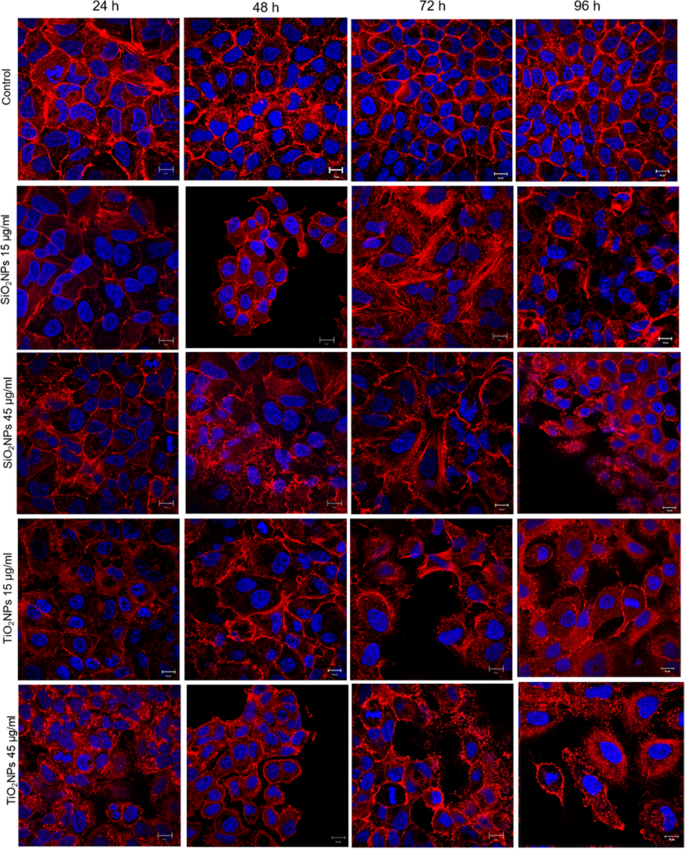
Effect of SiO2 NPS and TiO2 NPs on actin network on A549 cells. A549 were treated with 15 μg/ml and 45 μg/ml of NPs for 24 h, 48 h, 72 h, and 96 h; successively they were fixed and stained with Phalloidin–ATTO 488 and DAPI. The 2D images of cortical actin were acquired by a Zeiss LSM700 (Zeiss) confocal microscope equipped with an Axio Observer Z1 (Zeiss) inverted microscope using a × 100, 1.46 numerical aperture oil immersion lens. All data were processed by ZEN software (Zeiss)
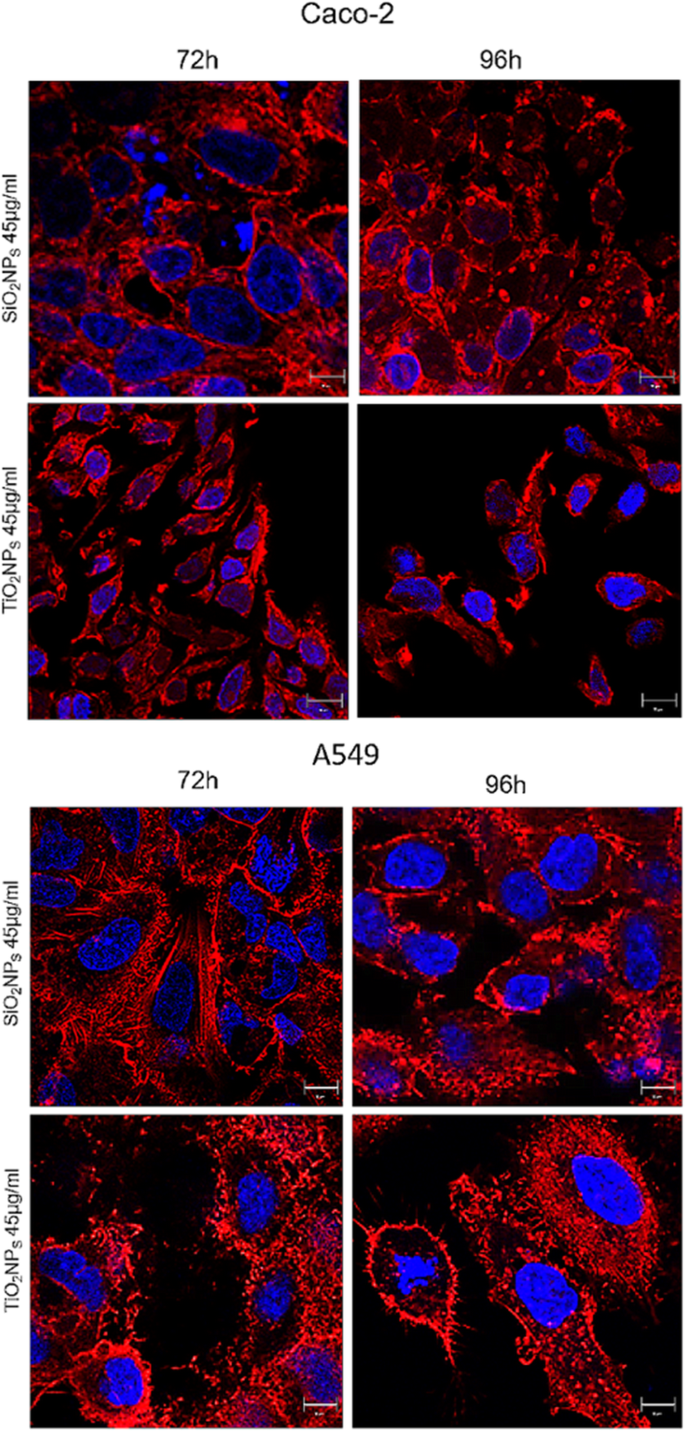
Local enlargement of confocal acquisitions acquired in Figs. 6 and 7 showed (more in details) the effect of SiO2 NPS and TiO2 NPs on actin network of Caco-2 and A549 cells after the exposure of 45 μg/ml of NPs for 72 h and 96 h
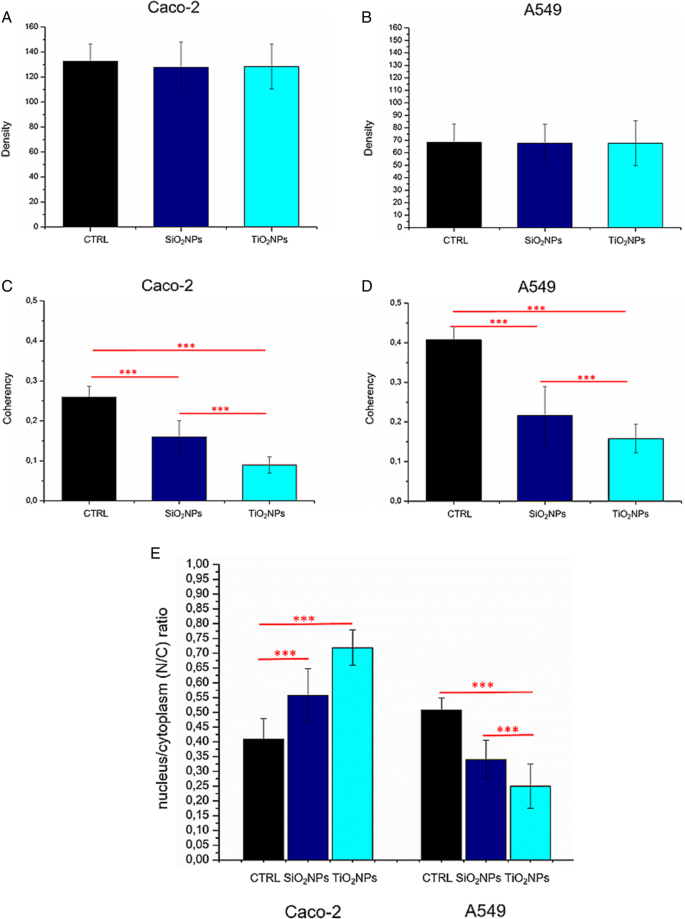
Integrated density (a 、 b ) and coherency (c 、 d ) for Caco-2 and A549 cells treated with 45 μg/ml of SiO2 NPとTiO 2 NPs after 72 h. The integrated density and coherency values were expressed as a mean value and relative SD, calculated from confocal acquisitions by ImageJ (calculation on 15 cells). The mean values and their standard deviations were reported in the histograms. Results were statistically significant for p < 0.05 (< 0.05*, < 0.01**, and < 0.005***). e Analyses of nucleus/cytoplasm ratio on Caco-2 and A549 after exposure to 45 μg/ml of SiO2 NPとTiO 2 NPs for 72 h. The ratio was calculated on 15 cells by ImageJ. The mean values and the SD were reported in the histogram. Results were statistically significant for p < 0.05 (< 0.05*, < 0.01**, and < 0.005***)
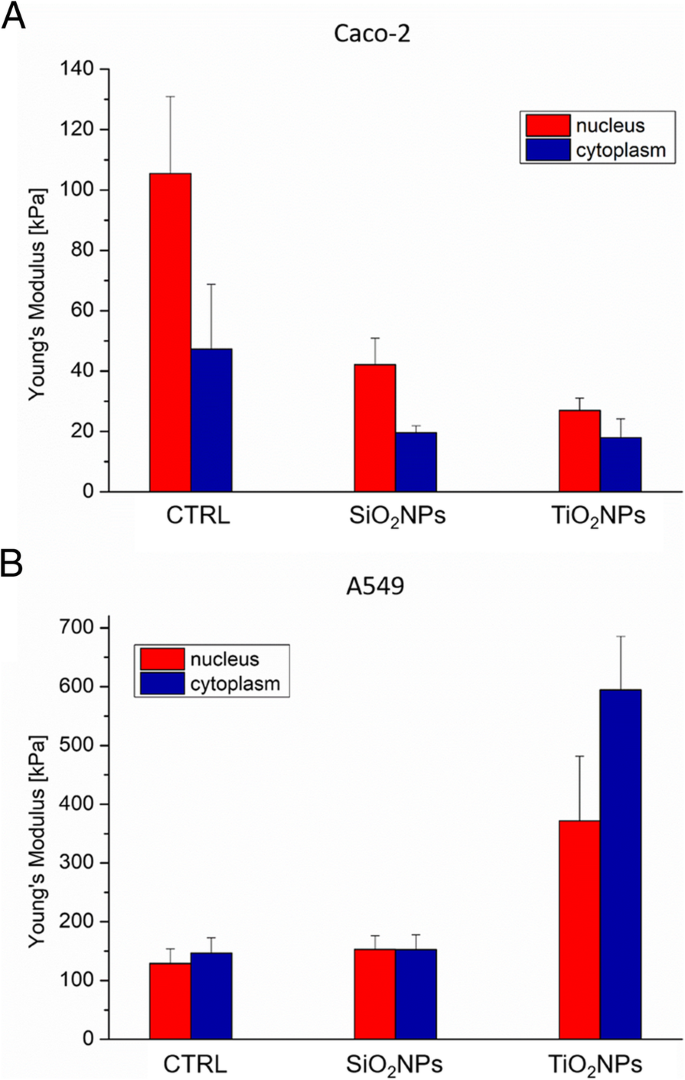
Young’s modulus values expressed in kPa, calculated from the nuclear region and the cytoskeletal area of Caco-2 (a ) and A549 (b ) cell lines after a treatment to 45 μg/ml of SiO2 NPとTiO 2 NPs for 72 h
Discussion
The spread of different kind of ENPs in several fields raises awareness about the importance to assess their potential toxicity in living organisms and the environments as well, taking into account their potential application in biomedical field [46,47,48]. In vitro and in vivo investigations are crucial to enrich the scientific knowledge and to release reliable clinical and epidemiological data [49]. The toxicity tests performed on different cells are considered the golden standard to assess the safety of NPs. However, few studies have investigated the interactions between NPs and cells from a biomechanical point of view. Cell mechanic is an important factor that influences many cellular pathways, including apoptosis, differentiation, migration, cancer metastasis, and wound healing [50]. In our work, we have addressed this point and related cell viability with the changes in mechanical properties of cells treated with different NPs. Firstly, we synthetized amorphous SiO2 NPs and crystalline TiO2 NPs with a size of c.a. 20 nm. NPs were stable in water and DMEM up to 96 h, even upon incubation with 10% and 20% of FBS. This was found to induce an increase in NPs size due to the formation of protein corona, in perfect agreement with the literature data. [51]。 Since the entry route of NPs often occurs through inhalation and ingestion, we opted to investigate the potential effects on Caco-2 and A549 cells, which are representative models for the intestinal tract and airways mimicking oral and inhalation uptake [52]. As primary investigation, we quantified the cellular internalization of SiO2 NPとTiO 2 NPs by elemental analysis. The most effective uptake was observed in Caco-2 cells, especially upon treatment with TiO2 NPs in a time-dependent manner. It has been reported that amorphous SiO2 NPs, with a small size range of 15–20 nm, can bind the plasma membrane and then passively pass across the lipid bilayer to get access into the cells [53]. As demonstrated in A549 [54] and Caco-2 [55], in fact, small SiO2 NPs can translocate in the cytoplasm with no apparent membrane encapsulation. The anatase crystalline form of TiO2 NPs is the more chemically reactive [56] showing a faster absorption with respect to rutile, as previously reported [32]. However, the uptake mechanisms of Caco2 are still unclear, despite that some hypothesis have been formulated, some of these include metal ion release upon NPs degradation in the intestinal barrier lumen or/and direct uptake by endocytosis. [57]。 In A549 cells, TiO2 NPs were localized in cytoplasm and close the nucleus region [58]. We used WST-8 assay to assess the influence of different concentrations of SiO2 NPs and TiO2NPs on cell viability. We have observed a general decrease of viability, especially in Caco-2, with TiO2 NPs displaying the strongest toxicity. After assessing the viability, we monitored the extracellular release of the cytoplasmic enzyme LDH. We confirmed that the NPs induced an extensive membrane damage, which relates also to the increase of intracellular ROS levels, resulting in oxidative stress. In this context SOD, which acts as strong antioxidant against ROS [59], was significantly reduced most probably because of the unbalance of the redox repair systems. In addition, the oxidative stress increased the lipid peroxidation [60], as demonstrated by MDA measurements after NPs incubation. This is particularly evident in Caco-2 cells after TiO2 NP exposure. It is worth mentioning that this effect can decrease membrane fluidity, which can further explain the observed higher entry levels of the TiO2 NPs [61]. This was in significant accordance with the intracellular oxidative stress levels measured by SOD inhibition, as well as with the reactive oxygen species generation. After these assessments, we investigated the modulation of the cell cytoskeleton, as an increase of intracellular ROS could affect the F-actin organization [62]. The cytoskeleton is characterized by a set of filaments (actin microfilaments, microtubules, and intermediate filaments) organized in a network that affects the mechanical properties of cells, as well as their behavior [29]. In particular, actin filaments are crucial for cell mechanics, and any alterations may induce aberrations in cell morphology under sub-toxic conditions [63]. It has been demonstrated that actin was one of the most commonly bound protein by SiO2 NPとTiO 2 NPs in cellular extracts. This definitely suggests that the actin functions, as well as cell motility and organelles trafficking, can be strongly affected by the presence of these NPs [64, 65]. As a further proof, several in vivo studies have revealed the potential of NPs to induce alterations in the expression of genes related to the cytoskeleton [63]. In order to understand how NPs modulate the cytoskeleton, we performed qualitative and quantitative confocal analyses on Caco-2 and A549 cells, after SiO2 NP and TIO2 NP治療。 We focused on actin stress fibers and cortical actin because they allowed to maintain the physiological mechanical architecture of cells. As reported in Figs. 4 and 5, the treatment with NPs induced a significant reorganization of actin. This was more evident after 72 h of treatment with 45 μg/ml of NPs, and especially with the use of TiO2 NP。 The adverse effects were stronger in intestinal cells, where we have observed the formation of protrusions and philopodia at the plasma membrane level, together with the disruption of tight junctions. Fluorescence coherency and fluorescence density have been used as quantitative parameters to assess alterations of actin distribution in the cytoskeleton. While coherency gives information about the organization of actin, density quantifies the levels of fluorescent actin. Caco-2 and A549 exposed to NPs showed a reduction of coherency compared to untreated cells, especially upon incubation with TiO2 NP。 This was in good agreement with the qualitative confocal imaging analyses. The fluorescence density of actin was not altered by NP treatment in both the cell lines, even if untreated Caco-2 cells showed higher values with respect to untreated A549. These data could suggest a potential difference in the amount of actin, which is dependent on>the specific cell type. We also evaluated the nucleus-to-cytoplasm ratio as the relative area of the nucleus over the whole cell. We confirmed a reduction of values in A549 and an increase of the ratio in Caco-2 with respect to the control cells. This indicates changes in cell morphology after NP treatment:Caco-2 underwent an increase of nucleus area, whereas A549 became larger through cytoplasm extension. As a final point, we explored any potential change in cell elasticity upon NP treatment. Cell elasticity is commonly expressed by Young’s modulus (E), which is a ratio between the stress and the applied strain (with unit in Pascal) [66, 67]. Changes in cell elasticity due to cytoskeleton reorganization is often associated to disease progression [68], hence (E) can be a refined indicator of potential pathological states [67]. The deformability of cells was measured through indentation experiments by AFM [69]. Many studies showed the detrimental effects of NPs on the F-actin that induced an enhancement of cell elasticity. However, a clear relation between change in cell stiffness, actin rearrangement and cell viability has not been clearly established yet. Here, we have covered such topic and found that Caco-2 and A549 cells significantly change their (E) upon NP treatment, even though in two different ways. Caco-2 cells are softer as confirmed by the decreased Young’s modulus, which has been found to be a function of both the NPs type and the cell regions analyzed. In particular, TiO2 NPs induced a general enhancement of elasticity, and this effect is more evident in the nuclear regions rather than the cytoplasmic one. On the other side, A549 displayed a remarkable increase of Young’s modulus after TiO2 NP exposure in cytoplasm region, compared to control cells (594 ± 40 kPa versus 146 ± 26 kPa, respectively). These data indicated that TiO2 NPs induce dose-dependent changes in force–deformation profiles in both cell lines, whereas SiO2 NPs showed little effects. The decrease of Young’s Modulus, and consequently an increase of elasticity after NPs exposure, can potentially impact cell homeostasis and physiological pathways. The reorganization F-actin, together with a reduction of coherency, showed a strong modulation of mechanical cell properties. NPs have been demonstrated to make the nuclear region of Caco-2 cells softer than untreated cells. The increase of elasticity (corresponding to a reduction of Young’s modulus) is a critical factor in tumor progression, because it is an indicator of disruption of cell junctions, which promotes in turn cell migration and metastatization [70]. Therefore, the treatment with NPs on Caco-2 (and TiO2 NPS in particular) can potentially promote migration due to change of elastic properties and deformability of cells. Also, the larger and softer nucleus area can be associated to cancer progression [71].
Conclusion
In this paper, we careful assessed the adverse effects of SiO2 NPとTiO 2 NPs on two different cell lines (Caco-2 and A549), mimicking the typical tissue that are exposed to NPs (ingestion and inhalation). SiO 2 NPs presented a low cytotoxicity in comparison with TiO2 NPS. We demonstrated how the cellular exposure to high doses of NPs induced morphostructural changes in term of actin reorganization, coherency, density and nucleus/cytoplasm ratio, which were more evident upon TiO2 NP治療。 Cell membrane deformability measurements showed different behavior in the two cells. In Caco-2, the cell elasticity increased after NP treatment, whereas A549 displayed an increase of stiffness. These results demonstrated that NPs induce modifications of cytoskeleton structures and, as consequence, a different Young’s Modulus values. Hence, the phenotype of cancer cells can turn into a more invasive profile, characterized by enhanced migration. On the other side, the increased stiffness observed in A549 might not promote the mobility of this specific cell indeed. We are sure that the analysis of cell mechanics upon NP exposure, combined with standard toxicological assays, will represent a golden standard to accurately assess the safety of NPs and to predict any potential possible diseases triggered by NPs.
略語
- A549:
-
Human adenocarcinoma alveolar basal epithelial cells
- AFM:
-
原子間力顕微鏡
- ATP:
-
アデノシン三リン酸
- Caco-2:
-
Human epithelial colorectal adenocarcinoma
- CLSM:
-
Confocal Laser Scanning Microscopy
- DAPI:
-
4′,6′-Diamidino-2-phenylindole
- DCF-DA:
-
2 '、7'-ジクロロフルオレセインジアセテート
- DLS:
-
動的光散乱
- DMEM:
-
ダルベッコの改良イーグル培地
- ENP:
-
人工ナノ粒子
- FBS:
-
ウシ胎児血清
- HCl/HNO3:
-
Hydrochloric/nitric acid
- ICP-AES:
-
Inductively coupled plasma atomic emission spectroscopy
- LDH:
-
Lactate dehydrogenase
- MDA:
-
Malondialdehyde
- NH4OH:
-
Ammonium hydroxide
- NP:
-
ナノ粒子
- PBS:
-
Phosphate Buffered Saline
- ROI:
-
関心のある地域
- ROS:
-
Reactive Oxygen Species
- SiO2NPs:
-
Silicon dioxide nanoparticles
- SOD:
-
Superoxide dismutase
- TBA:
-
Thiobarbituric acid
- TEM:
-
透過型電子顕微鏡
- TEOS:
-
オルトケイ酸テトラエチル
- TiO2NPS:
-
二酸化チタンナノ粒子
- TTIP:
-
Titanium (IV) isopropoxide
- XRD:
-
X線回折
ナノマテリアル
- 化学センシング用の貴金属ナノ粒子で装飾されたエレクトロスピニングポリマーナノファイバー
- HT29およびSPEV細胞株に対するAuナノ粒子の影響のinvitro研究
- 金属ナノ粒子からなる効率的な太陽熱吸収体の数値研究
- 抗菌剤として銀ナノ粒子で装飾された酸化グラフェンベースのナノコンポジット
- ラットにおける腹腔内および静脈内投与経路による生合成された銅および酸化亜鉛ナノ粒子の比較invivo精査
- 金属および金属酸化物ナノ粒子のグリーン合成と単細胞藻類Chlamydomonasreinhardtiiに対するそれらの効果
- 酸化亜鉛ナノ粒子の特性と微生物に対するそれらの活性
- ポリ(γ-グルタミン酸)は、Fe-Pdナノ粒子によるp-クロロフェノールの脱塩素化の促進を促進します
- 小さな希土類フッ化物ナノ粒子は、電気極性相互作用を介して腫瘍細胞の成長を活性化します
- 溶媒組成の調整による準安定相を持つCIGSナノ粒子の相選択的合成
- ナノ粒子の細胞取り込みと細胞内輸送への洞察