人体温度モニタリング用の印刷可能で高感度の柔軟な温度センサー:レビュー
要約
近年、フレキシブルセンサーの開発と研究が徐々に深まり、体温を監視するためのウェアラブルでフレキシブルなデバイスの性能も向上しています。人体の場合、体温の変化は人間の健康に関する多くの情報を反映しており、異常な体温の変化は通常、健康状態が悪いことを示します。体温は環境に依存しませんが、体表面温度は周囲の環境の影響を受けやすく、体温監視装置に課題があります。人体のさまざまな部分の温度をリアルタイムで高感度に検出するために、研究者はさまざまな種類の高感度の柔軟な温度センサーを開発し、電子皮膚の機能を完成させ、多くの実用的なアプリケーションを提案しました。この記事では、体温の変化を監視するために使用される高感度のパターン化された柔軟な温度センサーの現在の研究状況をレビューします。まず、フレキシブル温度センサーに一般的に使用される基板と活物質をまとめました。次に、柔軟な温度センサーのパターン化された製造方法とプロセスを紹介します。次に、温度測定範囲、感度、応答時間、温度分解能など、柔軟な温度検知性能について包括的に説明します。最後に、非常に繊細なパターンに基づく柔軟な温度センサーのアプリケーションが示され、柔軟な温度センサーの将来の課題が予測されています。
はじめに
健康な代謝には、代謝と比較的一定した体温に基づく人体のすべての生命活動が必要です[1]。高体温症または低体温症は、体内の酵素の活性に影響を及ぼし、それによって人間の代謝の通常の動作に影響を及ぼし、さまざまな細胞、組織、臓器の障害を引き起こし、重症の場合は死に至ることさえあります。体温の相対的な安定性は、体内の安定した環境を維持し、代謝などの生活活動の定期的な進行を確保するために必要な条件であることがわかります。人体の場合、体温の変化は人間の健康に関する多くの情報を反映しており、体温の異常な変化は通常、健康状態が悪いことを示します。人間の健康の監視[2、3]では、体温は無視できない重要な要素であり、体温のリアルタイムで正確な監視は特に重要です。
柔軟な温度センサーは、ウェアラブル、高感度、ポータブル、大面積、正確、そしてリアルタイムのトレンドに向けて開発されてきました。フレキシブル温度センサーは、主に温度変化による感熱材料の電気信号変化を利用して、温度のリアルタイム監視を実現します[4]。また、柔軟な素材の特徴を生かし、肌に密着して機能を発揮します。従来の温度測定器と比較して、持ち運びが難しく、高価で、監視の機会に適用できることに加えて、患者の意図的または意図的でない動き、および特定の場所(傷など]を監視できないため、制限があります[5 ]、体内の腫瘍切除部位[6])は、不正確または不完全な測定結果につながる可能性があります。上記の問題を解決するために、ウェアラブルで柔軟、薄く、高感度のパターン化された温度センサーは、科学研究者にとって研究のホットスポットになっています。
近年、体温を監視するための柔軟な温度センサーの研究が継続的に発展しており、多くの革新があります[7]。柔軟な温度センサーの大面積製造を実現するためのパターン化された製造の使用は、開発の傾向になっています[8]。自然界の生物学的構造を模倣することは優れたアイデアです[9]。吸着特性を備えたタコの足、生体温度の変化を感知できる毒蛇の頬[10]、一部の節足動物や哺乳類のひげのような構造[11]にも温度感知機能があります。センサーによって監視されている体温を明確に表示するために、研究者は柔軟な温度センサーを配置し[12、13、14]、イメージングデバイスまたはそのエレクトロクロミック材料を使用して熱イメージングマッピングを視覚化できます[5、15 、16]。柔軟な温度センサーに関する詳細な研究は、体温の高感度モニタリングなどの高い要件を満たすための適切な技術サポートも提供します。
この記事では、人体温度モニタリング、熱に敏感な材料、製造戦略、基本的なパフォーマンス、およびアプリケーションにおける高感度フレキシブル温度センサーの最近の研究の進歩をレビューします。最初の部分では、柔軟な温度センサーの材料を選択し、さまざまな柔軟な基板、柔軟な温度監視センサーとして使用できる感熱材料を要約します。第2部では、近年の文献での柔軟な温度センサーの使用に焦点を当てています。パターン化された製造方法をレビューし、典型的な製造プロセスを示します。第3部では、温度センサーの重要なパフォーマンスパラメーターを紹介します。第4部では、近年のフレキシブル温度センサーのアプリケーションシナリオと実際のアプリケーションを示します。最後に、印刷可能で高感度の柔軟な温度センサーの潜在的な課題と将来の開発の見通しについて簡単に説明します。
メソッド
資料
柔軟な基板
近年、電子技術や医療・健康分野での軟質材料の応用・研究が徐々に進んでいます。柔軟なセンサーを製造するには、センサー自体が柔軟で伸縮性があり、延性があり、センサーが依存する基板と回路が必要です。人体表面への接着に適応する特定の伸縮特性と伸縮特性、一般的な柔軟な基板は通常、ポリジメチルシロキサン(PDMS)[17,18,19,20]、ポリイミド(PI)[21、22などのフィルムに加工されます]、ポリウレタン(PU)[23]、ポリエチレンテレフタレート(PET)[24、25]、ポリビニルアルコール(PVA)[26]、ポリビニルブチラール(PVB)[27]、紙[28、29]、シリコーンゴム[5 、30、31]、およびペクチン[32]、綿、絹[33]、およびその他のセルロース材料[34、35]などのより皮膚に優しい生分解性材料も使用できます。
現在、フレキシブル温度センサーで最も使用されているフレキシブル基板はPDMSです。これは、比誘電率が2.3〜2.8、体積抵抗率が\({1} {\ text {.2})の優れた熱および電気絶縁材料です。 } \ times {10} ^ {{14}} \、\ Omega \、{\ text {cm}} ^ {-1} \)、その比比は\(1.03 \、{\ text {kg}} \ 、{\ text {m}} ^ {--3} \)25°Cでは、PDMSの熱安定性が高く、PDMSの熱伝導率は\(0.15 \、{\ text {W}} \、{\ text {m}} ^ {-1} \、{\ text {K}} ^ {-1} \)。[36]ガラス転移温度は125°Cと低く、熱膨張係数(CTE)[37]は\(301 \、{\ text {ppm}} \、^ {\ circ} {\ text {C}}です。 ^ {-1} \)。そして、ヤング率は\(\ approx 3.7 \、{\ text {MPa}} \)[38]であり、伸びとひずみが200%以上になります。その優れた伸縮性、拡張性、熱電特性[39,40,41]、高い化学的安定性、および使いやすさを考えると、PDMSは電子皮膚などの用途でより豊富になります[42]。 PDMS(表1に示す)と同様の特性を持つポリイミド(PI)材料である、PIは、\(0.1 \、{\ text {W}} \、{\ text {m}} ^ {のオーダーで熱伝導率が低くなります。 --1} \、{\ text {K}} ^ {-1} \)[43,44,45]、電気絶縁抵抗率\({1} {\ text {.5}} \ times {10} ^ {{{17}}} \、\ Omega \、{\ text {cm}} ^ {-1} \)[46]。比誘電率3.0〜3.6、ガラス転移温度が高く(360〜410°C)[47]、熱膨張係数(CTE)が低い(\(16 \、{\ text {ppm}} \、^ {\ circ} {\ text {C}} ^ {-1} \))。ヤング率≈\(2.8 \、{\ text {GPa}} \)[6、48]です。生体適応性、優れた伸縮性延性[49]、経済的、そして人体温度モニタリング用の温度センサーでの実用性[50]を備えたポリウレタン(PU)材料。薄膜フレキシブル基板は、優れた機械的特性を備えているだけでなく、優れた熱特性に基づくフレキシブル温度センサーの分野での応用研究にも適しています。図1に示すように、前述の有機ポリマー材料をフレキシブルセンシングに使用することに加えて、一般的なファブリックや、ファブリック[51、52]、シルク[53]、コットン[54]などの他の生分解性材料も柔らかく、変形可能、軽量、経済的、通気性、快適、耐久性、そして再利用可能。その他の利点として、柔軟な温度センサーのベース材料として研究することも期待され、懸念されています。
<図> 図>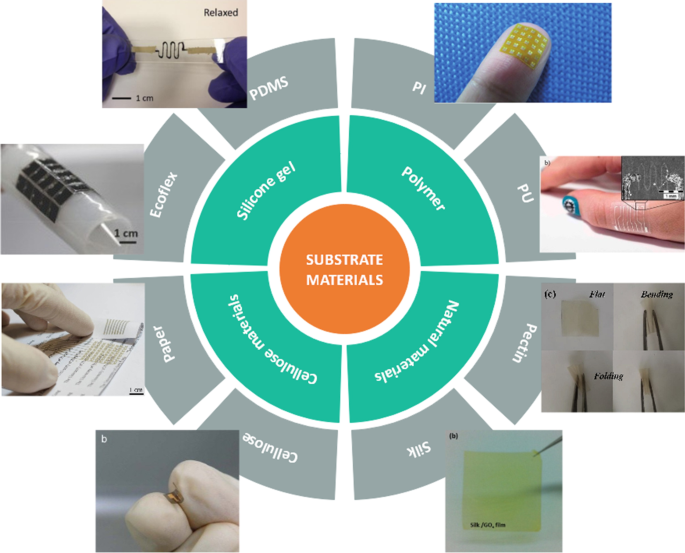
フレキシブルセンサーの一部の基板材料の概略図。右上から時計回りに:ポリイミド(PI)[55]、ポリウレタン(PU)[56]、ペクチン[32]、シルク[33]、セルロース[57]、紙[28]、エコフレックス[31]ポリジメチルシロキサン(PDMS)[ 58]
熱材料
活物質は、熱源と熱信号に直接かつ効果的に応答するセンサーに敏感であり、その特性は、温度に対する感度、応答時間の長さ、耐久性など、温度センサーの性能を直接決定します。温度に対する分解能[59]。製造が簡単で、原材料に適度で、生体適応性があり、ある程度の延性と優れた性能を備えた感熱材料は、柔軟な温度センサーの詳細な研究に大きな魅力があります[60、61、62]。
カーボン
さまざまな導電性フィラー(炭素ベースの材料、導電性ポリマー、金属粒子など)を半導体や絶縁性ポリマーのマトリックスに組み込むことにより、温度に敏感な導電性複合材料を作成するために多くの努力が払われてきました。一般的なカーボン材料には、カーボンブラック(CB)、グラファイト(Gr)、カーボンナノチューブ(CNT)、およびグラフェンが含まれます。
カーボンブラック(CB)とグラファイトは、その優れた電子的および機械的特性と低い処理コストのために、導電性フィラーとしてよく使用されます。その中で、CBはポリマーと混合して複合材料を形成するときに凝集体を形成しやすく、温度変化はその電気的特性に影響を与えます[63]。安定性により、温度抵抗係数(TCR)が高くなります[64]。グラファイトは炭素の同素体であり、優れた電気伝導率、熱伝導率、化学的安定性を備え、熱膨張係数は\(5.0 \ times 10 ^ {-6} \、{\ text {K}} ^ {-1}で小さくなります。 \)[65]。カーボンブラックと比較して、導電性フィラーとしてのグラファイト粉末は温度に敏感であり、2つとそれらの混合物は、充填されたポリマーによって形成される温度感受性材料複合フィルムのパーコレーションしきい値を低減します。膨張黒鉛(EG)は、天然黒鉛フレークから挿入、洗浄、乾燥、および高温膨張によって得られる、ゆるくて多孔質のワームのような物質である新しいタイプの機能性炭素材料です。材料が\(4.70 \、{\ text {W}} \、{\ text {m}} ^ {-1} \、{\ text {K}}に到達した後、ホットプレスによって形成された複合材料の熱伝導率^ {-1} {} \)EGの10 wt%がPDMSプレポリマーに含浸されている場合[66]。 Shih etal。 [67] PDMSをベース材料として使用し、グラファイト粉末を感熱検知材料として使用し、印刷プロセスでPIフィルムを使用して、Gr / PDMS複合センサーで構成される柔軟な温度センサーアレイを作成しました(図2aを参照)。従来のプラチナ(Pt)薄膜温度センサー(TCR =\({} 0.0055 \、{\ text {K}} ^ {-1} \))と比較して、周囲温度(30〜110°C)、グラファイトの体積分率が15%および25%の場合、複合材料のTCRは\(0.286 \、{\ text {K}} ^ {-1} \)および\ (0.042 \、{\ text {K}} ^ {-1} \)、結果は、Gr-PDMS複合材料の感度が高いことを証明しています。 Huangらは、グラファイトを充填したポリエチレンオキシド(PEO)とポリフッ化ビニリデン(PVDF)の複合材料を研究および製造しました[68](図2b)。シンプルなスピンコーティングプロセス。検出範囲が25.0〜42.0°C、高解像度が0.1°C、サイクル安定性が高く、優れた干渉防止能力(曲げ防止と防水を含む)を備え、1か月以内にセンサーを維持できる温度センサー。わきの下の温度を長時間連続して測定できます。その優れた機械的特性は、複合材料のフィラーとしてのGr粒子が、さまざまな曲率の下で移動しにくいためと考えられます。熱性能は、ポリマーの熱膨張に関連しています。一次元ナノ材料として、カーボンナノチューブが接続されて空間トポロジー構造を形成します[69]。直径は通常2〜20nmです。それは多くの異常な機械的特性(\(1 \、{\ text {TPa}} \)までの弾性率)、\(10 ^ {4} \; {\ text {S}までの電気伝導率を含む電気的特性)を持っています} \、{\ text {cm}} ^ {-1} \)[70]、固有のキャリア移動度(\({10,000} \、{\ text {cm}} ^ {2} \、{\ text {V }} ^ {-1} \、{\ text {S}} ^ {-1} \))[71]。近年、カーボンナノチューブやナノ材料の研究が深まるにつれ、それらの幅広い応用の展望も継続的に明らかにされています。カーボンナノチューブは優れた熱伝達性能を持ち、CNTはかなりのアスペクト比を持っているため、長さ方向に沿っています。熱交換性能は非常に高く、優れた設計アイデアや性能など、柔軟な温度センサーのアプリケーションも常に革新的です[72]。カーボンナノチューブとポリ(3,4-エチレンジオキシチオフェン)-ポリ(スチレンスルホン酸)(PEDOT:PSS)で形成された複合材料を調べたところ、多層カーボンナノチューブ(MWCNT)と単層カーボンの性能がわかりました。ナノチューブ(SWCNT)は同じではありませんでした[73]。同じ温度で、MWCNTとPEDOT:PSSによって形成された複合材料は、後で複合材料のインピーダンスを低下させる一方で、温度と湿度に対する感度も低下させます[74]。キムら[75]は湿式紡糸プロセスを使用し、PEDOT:PSSコンポジットも使用しました。 SWCNTを合成すると、複合材料の導電率と力率が大幅に向上し、複合材料の性能が向上します(図2d)。
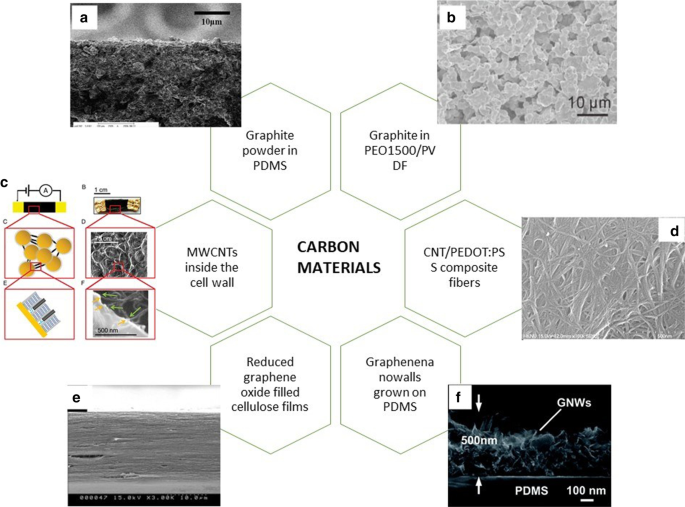
カーボン素材をベースにしたさまざまなフレキシブル温度センサー。 a グラファイト-PDMS複合材料のSEM画像[67]。 b PEO1500 / PVDF / Grの表面全体の顕微鏡写真[68]。 c サイバーウッドの概略図と走査型電子顕微鏡(SEM)画像[76]。 d CNT含有量が40wt%のCNT / PEDOT:PSS複合繊維のSEM画像[75]。 e CrGO100のSEM画像[54]。 f PDMS上のGNWの断面SEM画像[86]
生物学的材料を作成する従来の方法は、生物学的構造、生体工学、および生物学的インスピレーションのリバースエンジニアリングに依存しています。生物学的構造は、多くの場合、人工材料よりも優れています。たとえば、高等植物は高い応答性で温度変化を感知します。図2cに示すように、Giacomo etal。 [76]は、植物のナノバイオミメティック技術を使用して、単離された植物細胞とカーボンナノチューブ(MWCNT)で作られた優れた温度応答感度を備えたバイオミメティック材料を提案しました。細胞を直接接続するチャネルとしてCNTを使用し、有効温度抵抗率(TCR)が\(-\、1730 \%\、{\ text {K}} ^ {-1} \)のバイオニック材料を作成します。現在の最高のセンサーよりも2桁高く、監視温度範囲は35〜75°Cです。温度センサーに関する多くの種類の研究では、狭い温度範囲で高感度を達成できますが、広い温度範囲(40 K)では応答性能が不十分です。自然界のヘビのピット膜におけるヘビの生物学的温度感知構造も参照してください。生体膜は非常に高い温度と距離の感度を持っています。温血動物の獲物を特定の距離に配置するために使用できます。彼らはCa 2+ の追加を使用しました ペクチンフィルムへのイオンは、ヘビの頬フィルムの検知メカニズムを模倣して、CaCl 2 を添加したペクチンヒドロゲル温度感受性材料を調製します。 [10]。 <10 mKの感度で温度源をマッピングおよび監視し、特定の距離にある暖かい物体を感知することができます。植物細胞を組み合わせたり、動物の生物学的構造を模倣したりすることで、新しいアイデアが生まれ、柔軟なセンサーの研究の方向性がさらに広がります。
グラフェンは、六角形のハニカム格子を持つ2次元ハイブリッド炭素層です[77、78]。非局在化電子が多数存在するため、独自の電荷輸送により高いキャリア濃度が可能になります(\({10} ^ {{{33}}} \、{\ text {cm}} ^ {-2} \))、特定の環境条件下では、移動度が\({10} ^ {5} \、{\ text {cm}} ^ {2} \、{\ text {V}} ^ {{-{1}}} \、 {\ text {s}} ^ {-1} \)。そして、空間構造は豊富なビットドットを提供することができます。機能グループは、さまざまなアプリケーション要件を満たすように変更されます。同時に、高い流動性、優れた熱伝導率、優れた透明性、最大\(1 \、{\ text {TPa}} \)の弾性率、化学的安定性、および生体適合性を備えています[79]。さまざまな電子機器の応用分野で大きな注目を集めています[80]。優れた電子的および機械的特性を備えた透明な酸化グラフェン(GO)または還元型酸化グラフェン(rGO)は、GOの酸化またはさらなる還元後にグラファイト粉末によって形成された層状構造の製品です[81、82]。表面には、ヒドロキシル基、カルボキシル基、および環が含まれています。オキシ基などの多くの官能基は、簡単に変更でき、湿度、温度、化学物質などの環境条件、および目に見える応答特性に敏感です[54、83]。ただし、酸化グラフェン(GO)の低導電率は、電子デバイスには適していません。還元型酸化グラフェン(rGO)は、導電率を向上させるために熱還元によって合成されます。その優れた温度感度は、柔軟な温度センサーにも必要です[54、84、85]。グラフェンナノウォール(GNW)は、プラズマ化学気相成長法(PECVD)技術[77]とポリマー支援転写法を使用して、基板に垂直なグラフェンナノシートに成長します。成長プロセスは、より高いひずみ性能を持たせるために千鳥構造を形成します[61、83]。優れた機械的特性は、研究の温度センサーにも適用されています。電子皮膚アプリケーションでの多目的グラフェンとその誘導体の使用は、優れた性能、透明性、豊富な機能、および単純な製造プロセスを備えた柔軟な温度センサーへの道を開きます。
ヤンら。 [58]は、活物質として3次元プリーツグラフェンを使用し、リソグラフィーフィルタリング法[34]を通じて30〜100°Cの範囲の温度を監視できる柔軟な温度センサーを製造しました。ばねのような構造の特殊性により、温度変化の検出特性は最大50%のひずみで特徴付けることができます。歪みのない状態でのセンサーのTCR(\(-\、1.05 \ pm 0.28 \%\、{\ text {K}} ^ {-1} \))は、報告されているシリコンナノワイヤー温度センサー(\(0.15 --0.37 \%\、{\ text {K}} ^ {-1} \))回。この構造の熱材料の熱指数を調整できることは注目に値します。温度応答と回復は数十秒以内に完了することができるため、従来の硬質セラミックサーミスタと比較してその適用性が向上します。 Liu etal。 [87]は、温度に敏感な材料としてrGO材料を使用し、印刷技術によって軽量で低コストの柔軟な温度センサーを製造しました。監視温度は20〜110°C、感度は\(0.6345 \%\、^ {\ circ} {\ text {C}} ^ {-1} \)、応答時間は1.2秒に達する可能性があります。特定の応力およびひずみ特性により、特定の曲率面に取り付けることができます。同じ実験条件下で、還元型酸化グラフェン(rGO)、単層カーボンナノチューブ(SWCNT)、多層カーボンナノチューブ(MWCNT)を比較した後、直線性、感度、機械的特性、再現性を比較すると、 rGOを活物質として使用した温度センサーの性能は最もバランスが取れており、将来の電子スキンの大規模な準備のためのアイデアを提供します。 Sadasivuni etal。 [54]は、マトリックスとしてセルロースを使用し、フィラーとして熱rGOを使用して、温度変化に応じて25〜80°Cの柔軟で効率的な監視温度範囲を生成する複合フィルムを提案しました(図2eを参照)。容量性柔軟温度センサー線形関係で。標準の市販の白金温度センサーと比較して、温度センサーは金属腐食現象による汚染を長期にわたって引き起こさず、長期間安定性を維持することができます。 Trung etal。 [17]単純なスピンコーティング法とラミネーション技術により、透明で伸縮性のある(TS)フレキシブル温度センサーを製造しました。 rGOナノシートと弾性ポリウレタン(PU)基板で形成された温度検知層。複合材料の形成。電子デバイスの目新しさは、構造内の材料の各層がTSであり、透明で伸縮性のある基板上に簡単に直接コーティングできることです。そうすれば、人体に簡単に取り付けることができます。このデバイスは、0.2°Cという小さな温度変化を検出できます。 30%のひずみで10,000回伸ばされた後は、温度応答にほとんど影響を与えません。ひずみが70%の場合でも、デバイスを使用できます。ヤンら。 [86]プラズマ化学気相成長法(PECVD)技術を使用して、銅箔上に特殊なインターレース3D導電性GNWネットワーク構造を成長させ(図2f)、ポリマー支援転写法とPDMSを組み合わせて超高感度を形成することを提案しました。ウェアラブル温度センサー。熱応答は、従来の温度センサーの応答をはるかに上回っています。 GNWの優れた延性と熱感度とPDMSの大きな膨張係数により、センサーは25〜120°Cの温度変化を0.1°Cの精度、1.6秒の応答時間、8.52秒の回復時間で監視できます。 、および数か月以内に安定性を維持します。 TCRは\(0.214 \、^ {\ circ} {\ text {C}} ^ {-1} \)に達します。これは、標準の市販のプラチナ温度センサー(\(39.2 \ times 10 ^ { --4} \、^ {\ circ} {\ text {C}} ^ {-1} \))。柔軟な温度センサーでの炭素材料の使用は、健康監視、ウェアラブルデバイス、ロボット工学、人間と機械のインターフェース、および人工皮膚でのその応用の可能性を促進しました。
金属および金属酸化物
金属材料は一般に、金(Au)[88,89,90]、銀(Ag)[91、92]、銅(Cu)[93]、白金(Pt)[55、94]などの導電性材料です。図3c)、ニッケル(Ni)[95]、およびアルミニウム(Al)[96]。これらは主にセンサーの電極およびワイヤーとして使用されます。従来の剛性金属温度センサーと比較して、柔軟な金属温度センサーは機械的柔軟性が高く、高度に湾曲した表面に簡単に取り付けることができ、小さな温度変化や小さな範囲での分布を検出するのに適しています。現在の印刷プロセスに関する限り、一部の金属材料は温度に敏感で導電性が高く、導電性の金属ナノインク[97]、ナノフィラー[95]、ナノワイヤー[98]、および柔軟な温度センサーで広く使用されているアクティブな温度感受性層を作成するためのパターン化されたフィルム[19]。
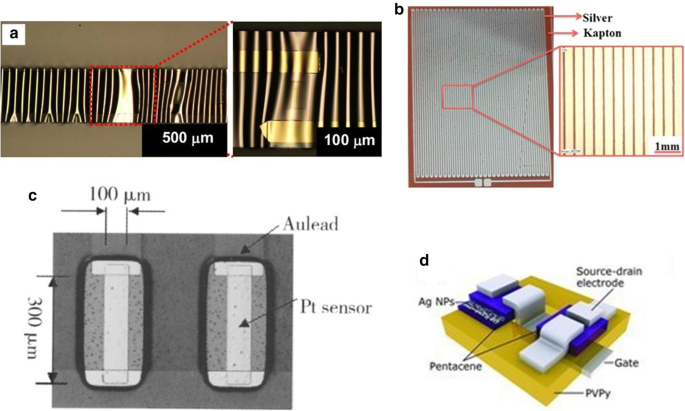
金属材料をベースにしたさまざまなフレキシブル温度センサー。 a 周期的に座屈したパターンを持つPDMS基板上の伸縮性センサー[99]。 b カプトン基板上のインクジェット印刷された銀温度センサーの写真[102]。 c 温度センサーの画像[6]。 d (1つのトランジスタ)–(1つのサーミスタ)温度センサーの概略図[101]
一般的な温度センサーで最も一般的な敏感な材料は、PtとAuです。ビン等。 [6]は、微小電気機械システム(MEMS)技術を使用し、剥離プロセス後にPIフィルム上に堆積する検知材料として白金を提案しました。必要なパターンを形成するラミネーションの後、20〜120°Cの温度変化を監視するために使用され、TCR値は\(0.0032 \、^ {\ circ} {\ text {C}} ^ {-1} \ )。高価なプラチナと比較して、Auはより優れた導電性と柔軟性を備えています。デバイスの性能が引張ひずみの影響を受けないことを実現するために、Yu etal。 [99]事前に伸ばされたPDMSフレキシブル基板であるクロム(Cr)/ Au薄膜(図3a)に巧みに使用されたスパッタ堆積、および可逆的な曲げ可能で伸縮可能なフレキシブル温度センサーは、フォトリソグラフィープロセスによって製造されます。デバイスの最大ストレッチが30%の場合、デバイスのパフォーマンスは変化しません。この研究は、フレキシブルセンサーの引張抵抗が低いという欠陥を改善します。 Dankoco etal。 [100]は、有機銀複合インクを使用して、インクジェット印刷によってポリイミドフィルムに銀の線を滑らかかつ均一に堆積させました(図3bを参照)。 20〜60°Cの測定可能な体表面温度は、曲がって柔軟な温度センサーになります。平均感度は\(2.23 \ times 10 ^ {3} \、^ {\ circ} {\ text {C}} ^ {-1} \)です。ただし、センサーのヒステリシスは<5%です。レンら。 [101]は、銀ナノ粒子(NP)/ペンタセンサーミスタと15〜70°の温度範囲の有機薄膜トランジスタ(OTFT)の統合に基づく、高熱分解能(ダイナミックレンジ=10ビット)の柔軟な温度センサーを提案しました。 C。この研究では、複合材料の温度依存性が高いことをテストし、サーミスタアプリケーションでの銀ナノ粒子(NP)の実現可能性を証明しました。ハイダイナミックレンジセンサーは、大面積センサーアレイや電子スキンにも適しています。チョン他[95]は、ニッケル粒子で満たされた半結晶性ポリエチレン(PE)とポリエチレンオキシド(PEO)ポリマーの混合物で設計された柔軟な温度センサーを開発しました。その中で、ニッケル粒子の濃度がニッケルフィラーの透過しきい値を超えると、高導電率(\(40 \、{\ text {S}} \、{\ text {cm}} ^ {-1} \))が可能になります得られる。二成分ポリマー混合物に特有の大きな正の温度係数(PTC)により、製造されたセンサーは、熱サイクルの安定性を維持しながら調整可能な高感度温度範囲を提供するだけでなく、再現可能な温度応答プロセスを実現します。センサーの感度は、標準の熱電対やワイヤレス伝送よりも3桁高くなっています(\(0.3 \、{\ text {V}} \、^ {\ circ} {\ text {C}} ^ {-1} \))。機能しますが、±3.1°Cの重大な誤差があります。ワイヤレスセンシングテクノロジーと印刷可能な素材を組み合わせて、幅広いアプリケーションを実現したいと考えていることは注目に値します。
金属酸化物も重要な活物質であり、温度センサーに広く使用されています。金属酸化物材料の高い抵抗の温度係数は、温度検知性能を向上させることができます。金属酸化物半導体の熱感度は、半導体化合物が温度によって変化し、抵抗値が変化する現象です。遼ら[103]は、無機熱材料である二酸化バナジウム(VO 2 )を含む高感度温度/機械式デュアルパラメータセンサーを報告しました。 )、転写印刷技術(VO 2 )によって製造されたPET /二酸化バナジウムに基づいています )/ PDMS多層構造。 VO 2 ポリマー支援堆積(PAD)技術によって堆積された層は、エッチングされ、PDMSフィルムに付着します。 270〜320Kの範囲の温度を検出できます。VO 2 の高いTCRに起因する0.1Kの分解能での温度検知性能 材料。体表面温度変化を正確にマッピングするために、Huang等。 [91]はインクジェット印刷プロセスを開発しました。酸化ニッケル(NiO)を使用して、PIフィルム上に安定したナノ粒子インクを生成します。小さな正方形のNiOフィルムが銀の導電性トラックの間の端に印刷され、温度センサーをすばやく製造します。曲げ試験でも性能を維持でき、熱電対と同様の応答速度を示します。金属と金属酸化物に関する広範な研究は、金属材料の熱特性に基づいたフレキシブル温度センサーの分野での金属材料の応用の基礎を築き、興味深い調査を示しました。
ポリマーと有機材料
ポリマーは、フレキシブルセンサーで最も使用されている材料です。基板または活性剤として使用されることに加えて、機械的柔軟性、軽量、透明性、安定した性能、容易な処理、および低い製造コストを備えた感熱性複合材料は柔軟性があります。温度センサーへの応用が注目されています。温度センサーでよく使用される感熱性ポリマーには、ポリ(3,4-エチレンジオキシチオフェン)-ポリ(スチレンスルホン酸)(PEDOT:PSS)[16、104、105、106]、ポリ(3-ヘキシルチオフェン)(P3HT)[107]、ポリピロール( PPy)[57]、ペンタセン[101]、ポリ(N-イソプロピルアクリルアミド)(pNIPAM)[108]、ポリ(フッ化ビニリデン)(PVDF)[109,110,111,112,113]など
伸縮性カーボンナノチューブ温度センサーの精度と堅牢性を向上させることができる回路設計戦略[114]に基づいて、Zhu etal。 [115]は、差動読み出し技術を使用して、OTFTに基づく伸縮可能な温度センサーのアクティブで高感度の層の組成を比較しました(図4aを参照)。その中で、アジド架橋を有するポリスチレン-ブロック-ポリ(エチレン-ラン-ブチレン)-ブロック-ポリスチレン(SEBS)およびポリ(ジケトピロロピロール-[3,2-b]チエノ[2 '、3':4,5]チエノ[2,3-d]チオフェン])(PDPPFT4)およびポリ(イソインジゴ-ビチオフェン)(PII2T)これら2つの有機半導体(OSC)は、ゲート誘電体上にブレンドおよびスピンコートされ、CNTは電極として使用されます。温度測定範囲は25〜55°Cです。内部では、2つのセンサーの温度係数は\(-\、2.89 \%\、^ {\ circ} {\ text {C}} ^ {-1} \)と\(-\、4.23 \%\、 ^ {\ circ} {\ text {C}} ^ {-1} \)、それぞれ。一軸ひずみ範囲が0〜30%の場合、誤差はそれぞれ<1°Cと<1.5°Cであり、差動読み出し方法の実現可能性と一般化可能性をさらに示し、伸縮性センサーのOSCについて説明します。横田ほか[116]は、半結晶性アクリレートポリマー/グラファイト複合材料に基づく大面積の超柔軟な温度センサーを報告しました。これは、複数のポイントで測定でき、印刷できます(図4b)。 25°Cから50°Cの間で、この温度で顕著な抵抗変化を示します。これは、人体の生理学的温度変化を測定するのに適しています。安定した熱サイクル安定性、最大20 mKの感度、100ms未満の高速応答時間を実現します。インビボ実験では、ラットの肺の中心温度の安定した変化が測定されましたが、センサーの高解像度は0.1°Cであることが証明されました。上記の特性に基づくセンサーアレイは、空間的な温度変化の動的な視覚的、熱画像のデモンストレーションを実現します。ただし、機器部品の通気性が悪く、長期摩耗が問題のひとつです。
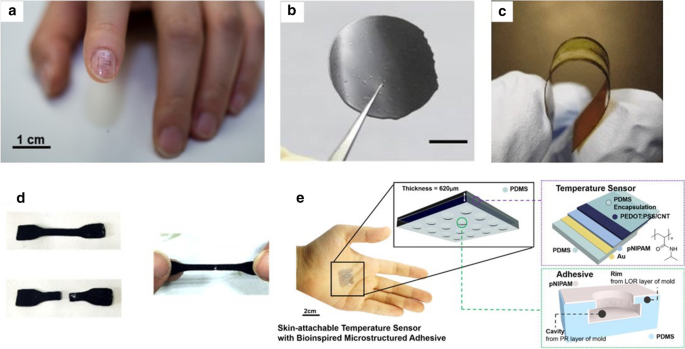
感熱性ポリマーをベースにしたさまざまなフレキシブル温度センサー。 a 爪に2つの温度センサーがあるサンプル[115]。 b グラファイトフィラーを含む共重合体のフィルムの写真(スケールバー、1 cm)[116]。 c 柔軟なカプトン基板上のTe-ナノワイヤ/ P3HT-ポリマー複合デバイスの写真[107]。 d DNヒドロゲルの自己修復プロセスの写真[121]。 e 手のひらの皮膚に取り付けられた製造された温度センサーの写真画像とタコを模倣した微細構造接着剤の全体的な概略図[108]
自己供給エネルギーは常に多くの人々の注目の的となっています[117]。柔軟な機器による自己供給エネルギーの実現は、機器の外部エネルギーの必要性を大幅に減らし、柔軟な機器をよりポータブルでより簡単にします[118]。その中で、熱電高分子材料は、必要な自己供給エネルギーの実現です。ヤンら。 [107]は、室温で成長させたTeナノワイヤーとポリ(3-ヘキシルチオフェン)(P3HT)ポリマーによって形成されたクリーンな複合熱電材料に基づく柔軟な熱電ナノ発電機(TENG)を開発しました(図4cを参照)。 TENGは、55 Kの温度差でのみ発電できます。熱電材料の特性により、TENGは、デバイス全体の温度差を監視するための柔軟な温度センサーとして使用でき、人間の体温をエネルギー源として使用できます。センサーに直接電力を供給します。室温での監視感度は0.15Kです。さらに、応答時間が0.9秒で、室温での最小温度変化が0.4Kの別のセルフパワー温度センサーも実証されました。温度分解能が小さいため、センサーデバイスは指先の温度変化を監視できます。自己供給により、柔軟なセンサー機器をより独立させ、重量を減らすことができます。将来的にはフレキシブルセンサーの開発も可能です(表2)。
<図> 図>低コストで環境に優しく、入手が容易で、優れた生体適合性を備えたプロセス材料は、人間が継続的に大量生産に対応するために追求するための必須条件でした[119]。そのようなほとんど無尽蔵の生物学的材料であるセルロースがあり、それは優れた特性を持っています。その弾力性およびその他の利点は、フレキシブルセンサーデバイスでも重要な役割を果たし、フレキシブル基板として使用できます。ポリピロール(PPy)は、優れた電気化学的安定性と迅速な応答を備えた線状の生体適合性ポリマーです。 Mahadeva etal。 [57]は、独自のセルロースとナノ厚のポリピロール(PPy)の優れた電気的特性を組み合わせて、温度と湿度に敏感な複合材料を形成する、その場での重合誘起吸着に基づく方法を報告しました。環境にやさしく、低コストで、生体適応性のある柔軟な温度センサーの製造に使用される材料。湿度に対する材料の感度のため、温度が上昇すると、センサーの静電容量も増加します。ヒドロゲルは、近年の研究で継続的に注目されています[120、121]。その優れた自己修復能力、優れた靭性と伸縮性、および生物学的適応性により、フレキシブルエレクトロニクス、健康監視、生物医学診断などのアプリケーション分野で大きな研究関心を呼んでいます[122、123]。ただし、優れたイオン伝導体としてのイオン性ヒドロゲルは、さまざまな刺激に応答でき、機械的強度が弱いヒドロゲル、および温度感度の低下は、柔軟な温度センサーを適用する際の課題となります。従来のヒドロゲルの欠点を解決するために、An etal。 [121]は、優れた温度センサーの自己修復特性を備えたダブルメッシュイオン伝導ダブルネットワーク(DN)ヒドロゲルを提案しました。 DNヒドロゲルの自己修復プロセスを図4dに示します。動的な物理的架橋と高伝導性の疎水性会合ネットワークおよびイオン会合ネットワークを備えたヒドロゲルへの高熱伝導率のカーボンナノチューブの添加は、DNヒドロゲルの温度感度を改善します。線形ヒドロゲル温度センサーは、複雑な物体の表面に完全にフィットし、敏感な抵抗変化を生み出すことができます。この材料の研究開発は、生物医学およびフレキシブルエレクトロニクスの分野でのヒドロゲルの応用を拡大します。
最近の研究では、PEDOT:PSSは、印刷可能な柔軟な温度センサーでよく使用される新しいタイプの有機導電性ポリマーです[124]。一般的に、PEDOT:PSSには、高導電率(\(10 ^ {3} \、{\ text {S}} \、{\ text {cm}} ^ {-1} \))、優れた熱電性能という利点があります。 [125,126,127,128]、強力な安定性[123、129]、およびドープ時の透明性[60]。ほとんどのポリマーはp型半導体です。ジメチルスルホキシド(DMSO)[130]やエチレングリコール[131]などのポリヒドロキシ有機化合物などの溶媒を加えることにより、ポリマーの導電率を数十倍、さらには数百倍に高めることができます。原田らは、CNTと導電性PEDOT:PSSソリューションのさまざまな複合比率を使用して、温度感度が\(0.25〜0.78 \%\、^ {\ circ} {\ text {C}}以内の一連の複合感熱フィルムを製造しました。 ^ {-1} \)さまざまな印刷プロセス[11、132、133、134]。検知性能は、一般的な金属温度センサーよりも優れています。一部のデバイスは、0.1°C未満の体温に近い解像度または90ミリ秒の高速応答時間を示しました。研究チームは、さまざまな印刷方法を使用して、さまざまな構造と優れたパフォーマンスを備えたさまざまな柔軟な温度センサーを作成しました。さらに、医療および健康のウェアラブルデバイスにおける柔軟な温度センサーのアプリケーションを強化しました。また、印刷可能で柔軟なセンサーについて考えるすべての人を刺激しました。印刷可能で柔軟な温度センサーの製造の詳細は、次のユニットで拡張されます。複合比を変更すると、複合フィルムの性能に影響を与えるだけでなく、構造の改善により、同じ複合フィルムの特性も最適化されます。図4eに示すように、Oh etal。 [108]フォトリソグラフィーストリッピングプロセスとスピンコーティングプロセスによって作られた生物学的材料を示し、タコの足吸盤の接着構造を刺激的に模倣しました。これは、ポリ(n-イソプロピルアクリルアミド)(pNIPAM)温度感受性ヒドロゲル、PEDOT:PSSおよびCNTで構成される高感度抵抗温度センサーです。このデバイスは、25〜40°Cの温度範囲で\(2.6 \%\、^ {\ circ} {\ text {C}} ^ {-1} \)の感度を持ち、皮膚の温度変化を正確に検出できます。 0.5°Cの。吸盤と同様の微細構造とその粘度により、デバイスは曲げに対してある程度の耐性があり、刺激がなく、長持ちし、再利用可能な結合効果があります。
ポリフッ化ビニリデン(PVDF)とその共重合体であるポリフッ化ビニリデン(P(VDF-TrFE))をベースにした、透明でスケーラブルなナノコンポジット電界効果トランジスタで、安定性が高く、機械的特性が強く、歪みが少ないため、圧力検知、ひずみ検知によく使用されます。 、および赤外線(IR)光検出デバイス[135、136]。興味深いことに、このタイプのデバイスは人体からの赤外線放射にも非常に敏感であることが研究者によって発見されたため、人体の生理学的温度変化を監視するために使用されると予測されています[137]。 Trungらは、以前の研究経験[113、138]に基づいて、rGO /(P(VDF-TrFE))複合センシング活性層をチャネルとして使用し、単純なスピンコーティングプロセス、統合されたPEDOT:PSSは調整可能な柔軟な電界を製造できます-エフェクトトランジスタ(FET)温度センサー[139]。フィルムの温度応答と透明度は、rGOの濃度と複合フィルムの厚さを変更することで調整できます(図5a)。センサーは、30〜80°Cの温度変化を監視できます。 0.1°Cの分解能と監視機能、および超高温応答を備えた優れた温度検知性能は、ソフトコア温度センサーの分野での焦電ポリマー材料の適用の実現可能性を検証します。同様に、ティエン等。 [112]センサーが相互干渉なしに圧力および温度信号を収集できることを実現するために、彼らは以前の研究に基づいて、電界効果トランジスタ(FET)センシングプラットフォームを使用して応答敏感層の材料を変更することを提案しました。ポリフッ化ビニリデン(P(VDF-TrFE))とBaTiO 3 の混合物であると結論付けました (BT)ナノ粒子(NP)は圧電として使用され(図5bを参照)、熱電ゲート誘電体とペンタセンは、フレキシブルセンサーが複数の刺激を受けている場合に、FETプラットフォームに直接統合された圧力熱抵抗の有機半導体チャネルとして使用されます。出力信号を出力し、ひずみ結合の信号干渉を最小限に抑えます。 FETセンサーは、ひずみと温度を同時に不均衡に提示する可能性があります。 FETセンサーアレイは、刺激に視覚的に反応することもでき、エネルギー消費量が少なく、故障が少ないという利点があります。これは、将来、電子皮膚の分野で大面積のマルチモーダルフレキシブルセンサーを適用できる可能性を示しています。広い領域で印刷および製造できる柔軟なマルチパラメータOFETデバイスは、生物医学的モニタリング、赤外線イメージング、および電子皮膚において優れたアプリケーションの可能性を秘めています。
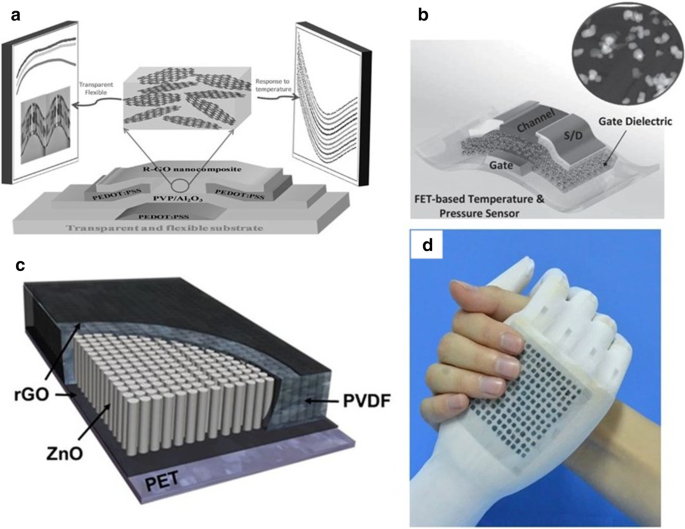
PVDF素材を含む柔軟な温度センサー。 a 透明で柔軟なrGO / P(VDF-TrFE)ナノコンポジットFETの概略図。回路図は、透明で柔軟なR-GO / P(VDF-TrFE)ナノコンポジットFETの構造的、光学的(透明)および電気的(温度への応答)特性を示しています[113]。 b ゲート誘電体がP(VDF-TrFE)とBaTiO 3 のナノコンポジットで構成されている、ボトムゲートおよびトップコンタクト構造の物理応答型電界効果トランジスタ(physi-FET)の構造 ナノ粒子とチャネルはペンタセンの有機半導体です[112]。 c ZnO / PVDF複合フィルムとrGO電極の概略図[110]。 d 柔軟なMFSOTEマトリックスの写真[23]
信号干渉を低減または排除するためのデカップリング方法に加えて、マルチパラメータフレキシブルセンサー信号の相互干渉の問題を解決するために、Lee etal。 [110]は、抵抗変化信号の回復時間に基づいて温度を推測する方法を提案しました。これにより、半導体酸化亜鉛(ZnO)ナノ構造が、フィラーとして基板のポリフッ化ビニリデン(PVDF)に混合されます(図5cを参照)。 )温度と圧力の信号を同時に収集できる高感度の多機能高感度層を作成します。その中で、半導体ZnOはPVDFの誘電率を高めることができ、熱安定性も備えています。張ら。 [23]は、セルフパワーの微細構造フレームでサポートされた有機熱電(MFSOTE)材料に基づくデュアルパラメーターフレキシブルセンサーを報告しました。図5eは、柔軟なMFSOTEマトリックスを示しています。温度と圧力の刺激によって引き起こされた信号の変化を2つの独立した電気信号に変換することにより、温度と圧力が同時に感知されます。このユニークな素材は、優れた温度検知特性を示します。モニタリング温度範囲は25〜75°Cです。分解能は<0.1 Kを達成でき、1Kの温度差での応答時間は<2sです。また、センシングのニーズに合わせてさまざまな基板に応じて調整することもできます。
製造
電子機器の柔軟性、多機能、簡単な製造、および高感度に対する要件の高まりに伴い、軽量、簡単なプロセス、低コスト、および大面積の製造を備えた柔軟なセンサー製造方法の探求と発見は、常に研究者が行ってきたものです。熱心です[140]。このセクションでは、主に、最近報告された柔軟な温度検知要素の製造戦略を要約し、それらのパフォーマンスを向上させるための主要なプロセスについて説明します。
薄膜の堆積
薄膜の作製方法は、使用する材料の相に応じて蒸着と相蒸着に分けることができます。相堆積には、後述するスピンコーティングおよびインクジェット印刷プロセスが含まれる。対照的に、蒸着は、蒸着プロセスに、物理蒸着(PVD)と化学蒸着(CVD)に分けられた化学反応プロセスが含まれるかどうかによって異なります。
PVDは、真空条件下で基板上に物理的方法で生成された堆積物または原子が薄膜を形成することであり、これは一般に電極または活性金属層を準備するために使用されます[141、142]。一般的な堆積方法には、真空蒸着、真空スパッタリング、およびイオンプレーティングが含まれます。その中で、金属ターゲットイオンスパッタリングは真空容器を指し、1500 Vの高電圧の作用下で、残りのガス分子がイオン化されてプラズマを形成し、陽イオンが電界の加速下で金属ターゲットに衝突し、導電性膜を形成するためにサンプルの表面にスパッタする金属原子[143]。 Ahmed etal。 [144]は、柔軟なPI基板に基づくSi温度センサーを導入しました。彼らは、高周波マグネトロンスパッタリングによって形成された金属電極間に検知材料としてドープされていないアモルファスシリコンを堆積させ、それらをパッケージ化した。最後に、温度検知素子は柔軟なポリイミドフィルムに埋め込まれており、検知性能には影響しません。 30°Cでの最大TCRは\(0.0288 \、{\ text {K}} ^ {-1} \)です。 Webb etal。 [145]は、明確で正確な熱性能モニタリングを提供するために、アレイの形で皮膚表面に自己組織化される2つの超薄型の皮膚のようなセンサー製造方法を導入しました。構造は、温度センサーアレイ、金属蒸着法、マイクロリソグラフィー技術、ウェットエッチング技術によってPIフィルム上に堆積されたCr / Au層の曲がりくねったトレース構造によって形成された敏感な層、および反応性接点と相互接続のためのイオンエッチングと金属蒸着がアレイを完成させます。別のセンサー構造は、多重化アドレス指定を使用して、ドープされたSiナノフィルムのパターン化されたPINダイオードセンサー設計を形成します。感度層は、金属蒸着、フォトリソグラフィー、化学蒸着、およびウェットエッチングステップによって定義されます。 2つのアレイを図6aに示します。アルミニウムフタロシアニンクロリド(AlPcCl)は、太陽電池や湿度センサーの材料としてよく使用されます。チャニらの研究開発中。 [146] AlPcClはサーミスタとして使用され、真空熱蒸発器を使用してガラス基板上のアルミニウム電極上に堆積されます。著者らは、AlPcClフィルムは、25〜80°Cの温度に対してより高い感度を示し、アニーリングによってセンシング性能を向上できることを発見しました。ビンらによって開発された柔軟な温度センサーで。感熱材料としてPt抵抗器を使用する[6]、スピンコーティングされたPIフィルム上に堆積されたAl層上にPtが蒸着され、Pt層は、敏感な層としてパターン化され、スピンコートされ、ポリイミド材料でパッケージ化されます。塩酸処理後、完全に柔軟な温度センサーが剥がされ、生物医学分野の物体の表面温度を測定するために使用できます。
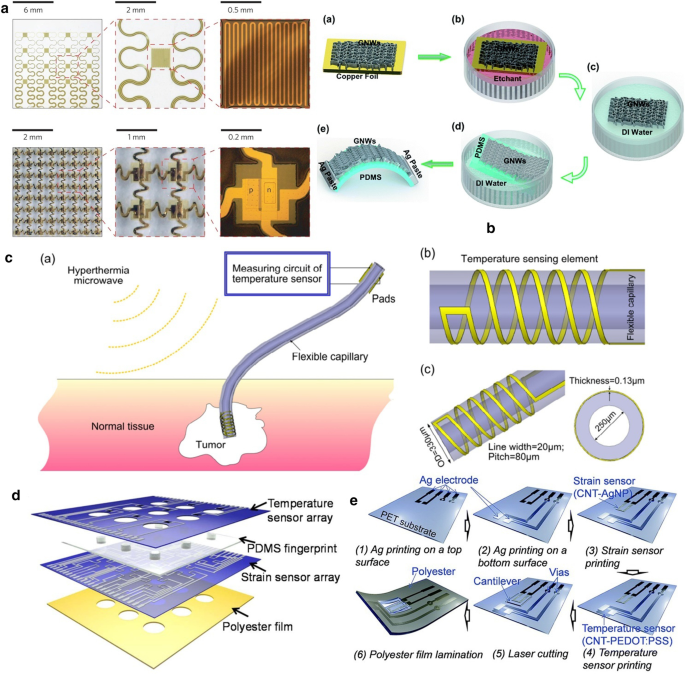
フレキシブル温度センサーの製造方法フレキシブル温度センサーの製造方法。 a 上:単一のセンサーの拡大図を備えた薄いエラストマー基板上に統合された4×4TCRセンサーアレイの光学画像。下:単一のセンサーの拡大図を備えた薄いエラストマー基板上に統合された8×8Siナノメンブレンダイオードセンサーアレイの光学画像[145]。 b GNW / PDMS温度センサーの製造の概略プロセス[86]。 c ポリマーキャピラリーへの埋め込み型マイクロ温度センサーのスケッチとその応用。ヘッドスパイラルセンシングエレメントは、フォトリソグラフィーによって製造されます[157]。 d e-skinデバイスの各層の概略図[132]。 e 印刷法を使用したPET基板上でのe-skin製造プロセスの概略図[134]
他の薄膜作製プロセスと比較して、化学蒸着法は高純度で高品質の薄膜を実現できます。原子層またはナノメートルレベルで構造化および制御できます[147,148,149]。低圧高周波プラズマ化学気相成長法(RF-PECVD)技術によって銅箔上にGNWフィルムを合成するプロセス。ヤンら。 [86] GNW / PDMSに基づく柔軟な温度センサーを開発しました。製造プロセスを図6bに示します。彼らは、GNWが温度センサーの活性層として実現可能であり、その熱応答性能が従来の金属温度センサーの性能を上回っていることを確認しました。従来のCVD技術と比較して、低温および低圧条件下でPECVD技術[150]を使用すると、堆積速度と膜品質を効果的に向上させることができます。別の研究では、周等。フローティング触媒化学蒸着(FCCVD)法[151]を使用して、制御可能な厚さの元のSWCNTフィルムを直接合成しました。この方法で成長させたCNTの連続ネットワークは、高い導電率と高い好ましいゼーベック係数を持っています。元のSWCNTフィルムをPET基板に転写した後、分岐ポリエーテルイミド(PEI)エタノール溶液をドロップキャストし、乾燥させて、フレキシブル熱電モジュールの製造に使用できるn型SWCNTフィルムを取得します。 CVDは任意の基板上に任意の材料を堆積させることができますが、シンプルで低コストで大面積の製造ナノデバイス製造技術の需要が高まり続けるにつれて、製造プロセスは複雑で高コストで有毒なCVD成長プロセスと時間のかかるエッチングプロセスは、より適切なフレキシブル電子デバイス製造技術に取って代わられています[58]。
マイクロナノパターン化された製造
薄膜パターニングは、フレキシブルエレクトロニクス製造のコアテクノロジーの1つです。これは、製造業で材料を上から下に除去するか、下から上に材料を追加するという基本的な考え方に従います。その主要な技術は、薄膜製造、パターニング、転写、複製、忠実度、およびその他の技術です。フレキシブルエレクトロニクスには、大面積、低温、低コストのパターニング技術が必要です。マイクロエレクトロニクスおよび微小電気機械デバイスのパターニング技術から学びました。しかし同時に、フレキシブル基板、有機材料、大面積などのフレキシブル電子デバイスの特性も考慮する必要があります。フレキシブル温度センサーで現在利用可能なパターニング技術には、リソグラフィー、印刷、ソフトエッチング、ナノインプリンティング、インクジェット印刷、レーザー焼結、転写印刷、ナノダイレクトライティング[152]、およびその他のプロセスが含まれます。
リソグラフィーは、フレキシブルエレクトロニクスでさまざまな独創的な幾何学的図形や構造を実現するためのパターン化手法です。フォトリソグラフィープロセスは、光の下で異なる感度と物理的および化学的反応を持つフォトレジストを使用することにより、フォトマスク上のパターンを基板に転写することを含みます。フォトリソグラフィープロセスは通常、絶縁体(通常はシリコンウェーハ)上のフォトレジストを使用して、スピンコーティング後に必要なパターンまたは構造をパターン化し、さらにストリッピングプロセスによってそれを実現します[153、154]。フォトリソグラフィープロセスとストリッピングプロセスにより、高い位置合わせとエッチングの精度、単純なマスク製造、および快適なプロセス条件により、通常、高精度で機能豊富な微細構造システムを実現できます。キムらによって開発された統合された温度センサーと熱アクチュエーターを備えた超薄型の柔軟な縫合糸の用途において。 [155]、彼らはフォトリソグラフィー技術を使用して機器をマイクロプロセスし、製造された柔軟な医療機器は安定した熱性能を持っています。利用可能な材料と精密機器の必要性によって制限されるリソグラフィー技術。加工可能な材料と薄膜の厚さには制限があります。多数の活物質を必要とするデバイス製造工程には適していません。ヤンら。 [156]は、柔軟な埋め込み型マイクロ温度センサーを提案し、表面マイクロリソグラフィーを使用して、ポリマーキャピラリーの外面にマイクロフレキシブル温度センサーをエッチングしました(ミニチュア温度計の検知原理図を図6cに示します)。敏感な材料としてPtを使用することは、優れた直線性を持ち、生物医学分野での埋め込み型温度センサーとして有望な未来を持っています。ただし、このテクノロジーはマイクロエレクトロニクス業界の基盤であり、ウェアラブルフレキシブルエレクトロニクスの時代を開拓しました。
科学技術の発展に伴い、印刷プロセスは従来のテキストと画像の分野からマイクロナノ構造のパターン化の分野に拡大しました。技術はさまざまな素材にさまざまな素材を堆積させることができ、印刷プロセスは環境に過酷ではありません。一言で言えば、印刷技術には活版印刷、リソグラフィー、グラビア印刷、スクリーン印刷が含まれ、ソフトエッチング[158、159]、転写印刷、ナノインプリント、およびその他の方法に進化しました。特定の実装方法に従って、ウェアラブルセンサーは、マスクの助けを借りて、印刷された部分と印刷されていない部分を区別することができます。マスク印刷では、転写するパターンを事前にデザインし、マスクを通して形成する必要があります。機能性の活物質は、機能性インクインプリンティングプロセス[160]を介して基板または電極に直接転写することができます。スクリーン印刷は典型的なマスク印刷技術です[161]。絶対圧による印刷工程では、機能性インクは、パターン化されたメッシュを備えたスキージを介して基板に転写され、パターンを形成します。独自の印刷方法により、スクリーン印刷により、平面または曲面での高速で大面積の低コストの製造要件を実現できます。これは、センサー動作回路、電極、およびセンサー検出要素の製造に広く使用されています。フォトリソグラフィー技術と比較して、スクリーン印刷はさまざまな材料にパターンを生成できます。ただし、そのパターン解像度は複雑な幾何学的形状の要件を満たすことができず、単純な形状のパターンを作成する場合にのみ適しています。横田ほか[116]さまざまな半結晶性ポリマーを攪拌してグラファイトと混合し、人間の生理学的温度モニタリング用の柔軟な温度センサー用の超柔軟な温度感受性コポリマーを形成します。超柔軟な温度センサー素子は、PIフィルム上に堆積された2つのインターデジタル金電極の間にグラファイトフィラーと混合されたコポリマーを挟み、次にホットプレスによって形成することによるマスク印刷によって印刷されます。ヤンら。 [58]は、平板吸引フィルター印刷法を使用して、マスクを通してグラフェンを堆積し、それを真空フィルターし、それを基板に転写して、3次元の折り畳みパターン構造を形成し、可変熱指数を持つ伸縮性グラフェンを生成しました。サーミスタは、センサーの検知領域と伸縮性を向上させます。事前に設計された伸縮性のある敏感な素材のパターンは、50%未満に伸ばされた場合でも、温度の敏感な監視を維持できます。より広い面積のセンサーを経済的に製造するために、原田らは複雑で費用のかかる製造プロセス(堆積やフォトリソグラフィーなど)を放棄しました。彼らは、印刷プロセスのみを使用して一連の多機能フレキシブルセンサーを製造することを選択しました。 PEDOT:PSS / CNT複合インクは、シャドウマスクを介してPET基板にスクリーン印刷することで形成された回路に印刷され、レーザー書き込み(LS)後にPDMSの下層と結合するための穴があります。指紋のような構造(図6dを参照)は、スクリーン印刷されたひずみセンサー層と組み合わされて、柔軟なセンサーアレイを形成します。接触接点による変形や温度差により、人間らしいモニタリング性能を発揮します。別の研究では、これも完全な印刷技術を使用して、Kanao etal。 [134]は、片持ち梁構造に基づく多機能の柔軟なセンサーアレイを提案しました(図6e)。彼らは、ひずみセンサーと温度センサーを柔軟なスクリーン印刷回路に配置しました。 PET基板の両面に、柔軟な温度センサー(PEDOT:PSS / CNT複合インク)を備えたパターン化されたシャドウマスクが、画面の電気接点プリント回路に印刷されています。人間の皮膚の感知特性を模倣するために使用される完全に印刷されたアレイセンサー。カンチレバービーム構造に歪みが生じると、熱源が基板の底面にある温度センサーに近づき、温度変化をより正確に監視します。
転写印刷は、パターン化された表面の凹状構造または凸状構造が、パターン化されていないスタンプを介して受容体基板に転写される印刷方法です。基本的な原理は、スタンプと基板に対して異なる粘度の印刷層を使用して、パターン転写を実現することです[162,163,164,165]。転写印刷には、直接転写印刷と間接転写印刷の2種類があります。柔軟な温度センサーの製造では、後者がよく使用されます。つまり、受容体基板に転写するために事前に印刷されたパターン化されたフィルムを使用します。前回のレビューでは、有機材料の多くの例が言及された敏感な層ですが、温度に敏感な材料として使用される無機材料はほとんどありません。 Liao etal。 [103]は、無機熱材料である二酸化バナジウム(VO 2 )を含む高感度温度/機械式デュアルパラメータセンサーを報告しました。 )、転写印刷技術(VO 2 )によって製造されたPET /二酸化バナジウムに基づいています )/ PDMS多層構造。 VO 2 ポリマー支援堆積(PAD)技術によって堆積された層は、エッチングされ、PDMSフィルムに付着します。延伸後、ナノタイプのクモの巣の亀裂が形成され、次に層が柔軟なPET基板に押し付けられます。 270〜320Kの範囲の温度を検出できます。VO 2 の高いTCRに起因する0.1Kの分解能での温度検知性能 材料。収集された温度信号と機械的信号は、アルゴリズムの違いによって分離され、温度と機械的変化の影響を同時に監視します。柔軟な温度センサーの不十分な伸縮性の問題を解決するために、Yu等。 [99]は、転写印刷技術に基づいて、フレキシブルデバイスを30%伸縮させてもセンサーの性能を維持できるフレキシブルデバイスを発明しました。彼らは、標準的なフォトリソグラフィー技術を介してSOI上にパターン化するサーミスタとしてAu / Cr層を使用しました。剥離した感熱層は、柔軟なPDMSスタンプを介して接着し、事前に伸ばされた柔軟なPDMS基板に転写および印刷して、基板を解放します。図7aに示すように、伸縮性のある柔軟な温度センサーを形成する製造プロセス。
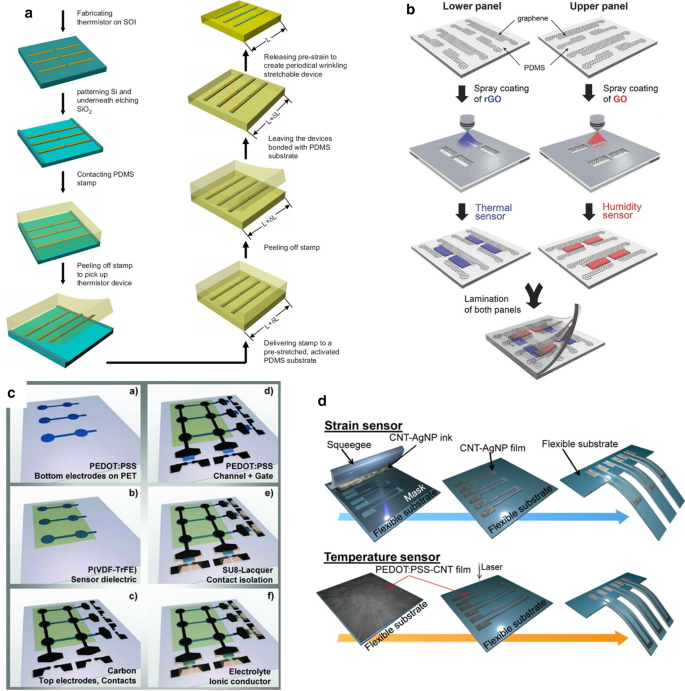
Fabrication method of flexible temperature sensor. a Schematic illustration of the fabrication process [99]. b Fabrication of the stretchable and multimodal all-graphene E-skin sensor matrix [84]. c Process flow illustrating the fabrication of printed ferroelectric active matrix sensor arrays [169]. d Multifunctional e-whisker fabrication [11]
Inkjet printing is an accurate, fast, and reproducible thin-film fabricating technology, which has been widely used in sensor development. Compared with other printing methods, inkjet printing has the advantages of convenience, flexibility, rapidity, low cost, compatibility, accuracy, etc. [166,167,168]. The patterns of inkjet printing need to be post-processed (drying, curing, sintering, etc.) to be fully formed. Improve the performance of printed patterns by converting ink nanoparticles into continuous materials. The properties of the surface tension and viscosity of the ink during the printing process, the quality of the printed pattern also places high requirements on the performance of inkjet equipment [97]. Under the condition of a specific size of the substrate, the conductive track's length formed as long as possible, and the thickness, width, and spacing of the track are reasonable. Repeated experiments obtain the ejection coefficient of the inkjet system. For example, Dankoco et al. [102] used the ink printing method deposit a composite ink with silver as the main component on the PET film to make a flexible and bendable temperature sensor. The circuit on the substrate is clear and smooth, and the ink drops are consistent, which used to measure human body temperature. The picture shows the fabricating process of the extremely sensitive and transparent multifunctional electronic skin sensor matrix developed by Oh et al. [108] The flexible array has the function of monitoring temperature, humidity, and strain. It can feel sensations, such as breathing and touch. GO and rGO, which used as humidity and temperature sensing materials, are sprayed on the PDMS substrate of the graphene circuit grown by the CVD method through inkjet printing technology through a mask. The two sensors are horizontally and vertically aligned, and the temperature sensor is on the bottom layer (as shown in Fig. 7b). After cross-lamination, a PDMS/graphene pressure strain sensor is formed. As a multifunctional flexible sensor, it can collect at the same time but independently respond to a single signal. Inkjet-printed graphene is seven orders of magnitude higher than CVD-grown graphene. The performance advantages reflected in many articles, and some research results are better than CVD-made graphite products. Inkjet printing and screen printing are both rapid and low-cost technical means to realize large-area sensor fabricating. Zirkl et al. [169] combined the two rapid fabricating technologies to create a fully printed flexible sensor array that uses multiple screens. In printing and inkjet printing, only five functional inks used to easily integrate multiple functional electronic components (including pressure and temperature-sensitive sensors, electrochromic displays, and organic transistors) on the same flexible substrate (in Fig. 7c). Because the fabricating speed and low cost of the process can also be applied to the smart sensor network using the roll-to-roll (R2R) fabricating process in the future. The development of a disposable electronic skin system (EES) is particularly critical. Similar to the previous example. Vuorinen et al. [56] introduced a temperature sensor similar to a band-aid after inkjet printing. The sensor uses graphene/PEDOT:PSS composite ink and the printing done on PU material suitable for skin. In particular, in addition to being able to achieve a sensitivity higher than 0.06% \(^{ \circ } {\text{C}}^{ - 1}\), they used inkjet printers to perform serpentine patterned inkjet printing between the silver screen printed with a high resolution (1270 dpi) improved the lack of inkjet printing for printing complex graphics such as snakes. With the use and research of inkjet technology in flexible electronics, the technology for controlling nozzles and inks is also improving. Compared with the speedy fabricating process of screen printing, inkjet printing needs to go through a debugging process, and the printing speed is not as good as screen printing. Also, the small number of nozzles running simultaneously and the high nozzle failure rate limit the inkjet printing fabricating technology to laboratories, and the large-area fabricating requirements of industrial production cannot achieve.
Laser direct writing (LDW) technology uses calculations to design pre-designed patterns. It directly uses laser beam ablation without masking and vacuums deposition. It can directly complete pattern transfer on the surface of the substrate material, with good spatial selectivity and high direct writing speed and processing accuracy, short cycle, high material utilization, and low pollution. Compared with traditional temperature thermistors that require high temperature and a variety of complex processes to activate the sensing function, LDW can achieve selective annealing on a predetermined pattern. A novel integral laser induced by Shin et al. [170] The laser-induced reduction sintering (m-LRS) fabricating scheme can also reduce metal oxide NPs during the annealing process. Scratch NiO NPs ink on a fragile PET substrate, and use m-LRS technology to directly reduce NiO to pattern a linear Ni electrode to form a planar Ni–NiO–Ni heterostructure (as shown in Fig. 8a). The unique method of fabricating a complete flexible temperature sensor system composed of Ni electrodes and NiO sensing channels from a single material NiO NPs provides a new idea for the rapid fabricating of flexible temperature sensors. Different from the previously designed and fabricated fully-printed sensor arrays, Harada et al. [11] proposed a more direct method of mass-fabricating flexible temperature sensors based on previous research. The flexible temperature sensing composite ink printed on the substrate, using the laser for etching away the excess part directly, leaves the designed pattern on the substrate and completes the fabricate of the temperature sensor array. Cut the base to imitate the animal's whiskers to create an artificial electronic whisker (e-whisker) structure (Fig. 7d), strain sensor formed by laminated screen printing. Bionic sensor arrays can scan and perceive three-dimensional objects by using structural advantages.
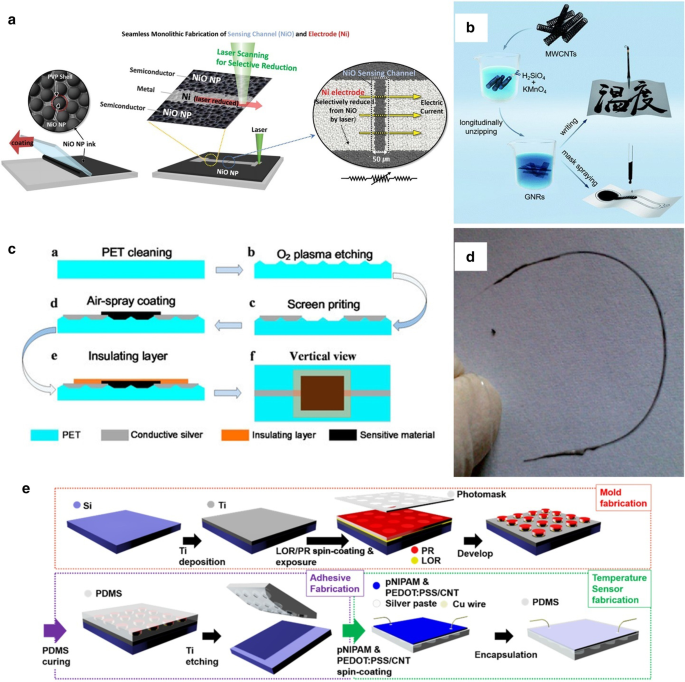
Commonly used fabricating methods. a Schematic of the m-LRS process [170]. b Schematic diagrams showing fabrication process of the paper-based GNRs sensors [171]. c Fabrication process of the temperature sensors [87]. d Flexible temperature sensor on yarn, contacts made of polymer conductive paste [111]. e Fabrication process for the mold, adhesive, and temperature sensor [108]
Under the premise of realizing low-cost and large-area fabricating, disposable intelligent monitoring equipment not only allows monitoring behavior to use anytime and anywhere but also ensures the safety and hygiene of the flexible monitoring equipment. Gong etal。 [171] proposed a pen-on-paper fabricating method that uses a brush write or a mask to graphene nanoribbon (GNR) conductive ink dripped between the carbon nanotube electrodes on the paper base. Shown in Fig. 8b. The flexible temperature sensor fabricated after encapsulation with transparent tape. In their research, GNRs have excellent sensing performance and meet the requirements for body temperature monitoring. The fabricating method provides reliable support for the application of fast, large-area disposable flexible equipment.
Other Commonly Used Fabricating Methods
Spin-coating the base layer, active layer, and encapsulation layer by layer is a common way to fabricate flexible temperature sensors by the liquid phase method. Spin coating technology often used for substrate fabricating and packaging protection. For solution-like sensitive materials, the spin coating used. The coating can not only significantly improve production efficiency but also has excellent potential for large-area fabricating.キムら[172] demonstrated the fabricating process of an organic field-effect transistor (OFET) array, using a spin-coating process to design a microstructure on a mold with a crystallized P(VDF-TrFE) material as a gate dielectric and other materials. Material packaging forms OFETs, and the introduction of microstructures enhances the sensing performance. The fabricating process of the bionic structure's [108] flexible temperature sensor fabricated. The adhesive layer of the bionic octopus foot sucker structure is fabricated by a spin coating process, combined with photolithography peeling technology and inverted mold technology (in Fig. 8e). It has an excellent temperature monitoring effect, and the materials used also perform well in terms of human biocompatibility. Liu etal。 [87] After surface ionization treatment on the substrate, the flexible electrode was screen-printed, and then the sensitive layer rGO was connected to the flexible wire through the manufacturing method of air-spray coating, and the main structure of the temperature-sensitive flexible sensor was formed after packaging (see in Fig. 8c). It is possible to make robot skins. Huang etal。 [68] fabricated a temperature sensor with flexibility, high resolution, and high repeatability in the temperature range of 25–42 °C. They dropped the PEO1500/PVDF/Gr composite solution after stirring and ultrasonic treatment on a polyimide flexible substrate. It was spin-coated at a certain speed to form a layer. It packaged it into a temperature sensor, which verified the medical body temperature transmission sense of possibility and feasibility. Spin-coating technology as an effective fabricating process for wearable devices has also introduced into Wu et al. research [173]. They designed an organic thin-film transistor with heat-sensing ability. Cleverly through the unique three-arm three-dimensional composite polylactide, polylactic acid (tascPLA) solution is spin-coated on the Au gate electrode evaporated on the Si substrate. Then the Au source and drain are deposited on it by a thermal evaporation method. The composite film containing tascPLA also serves as the gate of the OFET dielectric and substrate materials. In flexible electronics, there are not a few that use fabric as the carrier of the sensing element. The dip-coating of conductive yarn/fabric in conductive ink is the most commonly used coating technology for flexible sensors. The development of conductive fabrics provides a reasonable prerequisite for the application of smart fabrics. Sibinski et al. [111] developed a temperature sensor that monitors the temperature range of 30–45 °C. Moreover, it currently realizes filamentary miniaturization on a single yarn. In Fig. 8d shown, the PVDF monofilament fiber is coated with PMMA polymer compound mixed with multi-walled carbon nanotubes (MWCNTs) as a heat-sensitive layer by dip coating technology has good temperature sensitivity and extremely high repeatability. It is used as a fabric easily integrated into knit clothing. Another method, based on the molding properties of organic materials, is also used to fabricate flexible sensor membrane structures or to fabricate samples for testing sensing performance. The fabricating process of the thermoelectric nanogenerator (TENG) studied by Yang et al. [107] The drop-casting film method, after comparison, discards the PEDOT:PSS material, which has a weak drop-casting effect and is easy to break, not only the mixture of Te-nanowires and P3HT polymer in chlorobenzene solution is drop-casted on the flexible Kapton substrate to make a composite film, the composite material is also cast on the white fabric cloth, which also has apparent temperature sensing ability and thermoelectric performance, which can be used in wearable heat collectors. Rich and diverse fabricating methods lay the foundation for the development of flexible electronics. Under the requirements of large-scale and large-scale production, suitable fabricating technology is also continually developing. In the future, perfect wearable flexible devices are expected.
結果と考察
Key Parameters of Flexible Temperature Sensor
The flexible temperature sensor can be attached to the human body to monitor the subtle temperature changes on the surface of the human body or real-time temperature monitoring of specific parts, and even the temperature of the core or tissues and organs in the body. They respond to temperature changes by changing the resistance and output the temperature changes as electrical signals. With the increasing demand for flexible electronic devices, the disadvantages of traditional temperature sensors, such as poor scalability, inability to carry, and poor real-time performance, are becoming increasingly unsuitable for flexible wearable devices. Today flexible temperature sensors are required to have high performance, such as high sensitivity, fast response time, reasonable test range, high precision, and high durability to realize the monitoring function better.
Sensitivity
Sensor sensitivity refers to the ratio of the change ∆y of the system response under static conditions to the corresponding input change ∆x , that is, the ratio of the dimensions of output and input. When the sensor output and input dimensions are the same, the sensitivity can be understood as the magnification [21, 174, 175]. The temperature coefficient of resistance TCR (TCR, in \(^{ \circ } {\text{C}}^{ - 1}\)) of the common resistance type flexible temperature sensor is expressed in the following expression, \(\Delta R/R_{0} =s\left( {T - T_{0} } \right)\), is the relative resistance change (\(\Delta R/R_{0}\)) as a function of temperature, where \(s\) represents TCR. If the sensor output and input show a linear relationship, the sensitivity is constant [83]. Otherwise, it will change with the input quantity. Generally speaking, by increasing the sensitivity, higher measurement accuracy can be obtained. Most flexible sensors used for body temperature monitoring only pay attention to temperature changes within 10 °C and therefore require high-temperature sensitivity to capture relatively small temperature changes. Here, several typical methods and concepts for improving the sensitivity of flexible temperature sensors are summarized to provide a favorable reference for further improvements.
The sensing performance of flexible temperature sensors is usually closely related to the properties of materials. One possible way to realize the development of sensitive sensors is to adopt composite materials with visible thermal performance. After temperature stimulation, the internal conductive network will change, which will lead to affect the temperature sensitivity of the device. To improve the temperature sensitivity of the active material, a strategy that converts temperature fluctuations into mechanical deformation is adapted to amplify the response of the conductive network to temperature changes. The thermosensitive material is connected to a substrate with a high positive thermal expansion coefficient to enhance thermal induction deformed. This method has applications in sensors made of graphene, graphite and graphene-nanowalls. For example, in the flexible temperature sensor designed and fabricated by Huang et al. [68], Gr establishes a conductive path in the PEO/PVDF binary composite material. In the process shown in the Fig. 9b. The temperature change will cause the PEO to transform from crystal to amorphous, and melt when the temperature is close to the melting point of PEO, the volume expansion of PEO will destroy the conductive network in the composite material, resulting in a sharp increase in resistivity-increasing the strength of the PTC, which can quickly reaction within a narrow temperature range change. Experiments show that PEO thermal performance plays a leading role in the PTC effect of the device. Another way to improve the sensitivity of a flexible temperature sensor is to use a unique structure. In recent years, to improve temperature sensing performance, various design strategies have been developed by changing the structure. Yu etal。 [176] recently proposed a method based on engineering microcrack morphology to change the crack morphology of the PEDOT:PSS film on the PDMS substrate by adjusting the substrate surface roughness, acid treatment time, and pre-stretching degree to improve the temperature sensitivity of the sensor. Figure 9d shows the effect of average crack length and cracks density on temperature sensitivity. The result is that the longer the crack length, the higher the crack density, and the higher the temperature sensitivity. It is proved that the micro-crack structure plays a vital role in the temperature sensitivity of the sensor. It is verified that obtaining a high-density micro-crack structure is the key to obtaining the high-temperature sensitivity of the sensor. High density and high length directly correspond to higher temperature sensitivity. The fabricating process of flexible electronic equipment plays a vital role in the production of the device and can effectively improve the sensitivity of the flexible temperature sensor. Just as Shin et al. [170] used a rapid overall laser-induced reduction sintering (m-LRS) method for fabricating Ni/NiO flexible temperature sensor is different from the inkjet printing method. They directly reduce and sinter the Ni electrode on the NiO layer, and form a high-quality overall contact between the metal electrode (Ni) and the temperature-sensitive material (NiO). Ni–NiO–Ni heterogeneous temperature sensor shows higher temperature sensitivity than other sensors of the same type (Fig. 9e). Raman spectroscopy and X-ray diffraction (XRD) measurements show that the superior sensitivity comes from the unique thermal activation mechanism of the m-LRS process. Besides, since flexible temperature sensors are mostly touch-sensitive, it is inferred that the film's thickness affects the sensitivity. The study of Lee et al. [177] verified the effect of the thickness of the highly sensitive paper base on the sensitivity, they use a simple dipping fabricating method to deposit sensitive materials on the printing paper. Dipping time will affect the thickness of the film. The Fig. 9f also shows the different performance of sensitivity due to different thicknesses. It should be noted that the higher the sensitivity, the narrower the measurement range and, worse, the stability. Therefore, it is necessary to pay attention to increasing stability and accuracy while improving sensitivity.
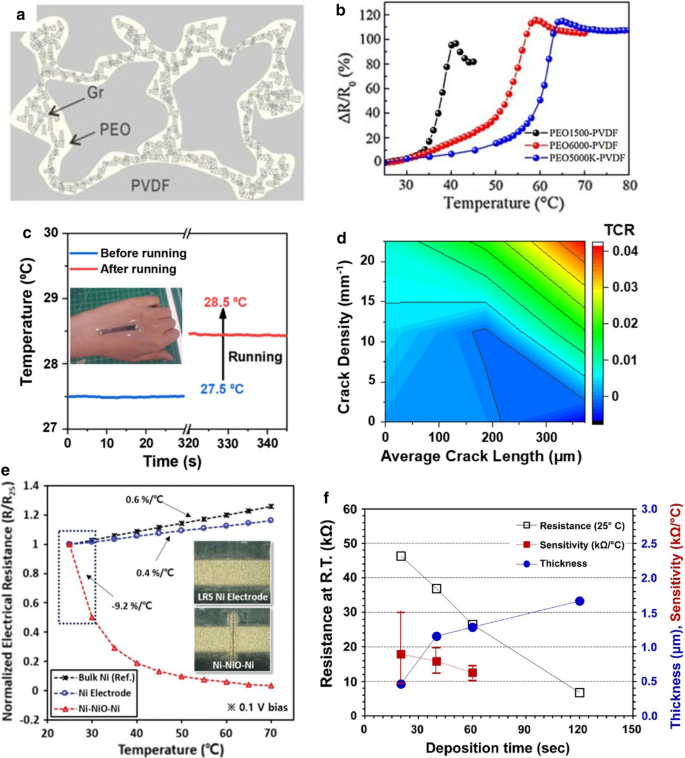
Sensitivity of flexible temperature sensor. a Images of the PEO1500/PVDF/Gr at room temperature. b Resistance curves of PEO1500/PVDF/Gr, PEO6000/PVDF/Gr and PEO5000K/PVDF/Gr composites versus temperature [68]. c The temperature measurement results before and after exercise, the illustration shows the flexible temperature sensor attached to the back of the hand. d Heatmap as functions of average crack length and crack density for TCR valuses from all developed sensors [176]. e PTC and NTC characteristics of the m-LRS processed Ni electrode and Ni–NiO–Ni structure. Inset digital images are m-LRS processed Ni electrode (upper) and Ni–NiO–Ni structure (lower) [170]. f The results of the deposited film thickness (blue circle), resistance at room temperature (white square) and sensitivity (red square) as a function of deposition time [177]
Other Parameters of Flexible Temperature Sensor
The sensing range of a flexible temperature sensor is an important parameter, which refers to the minimum and maximum temperature that can be detected. In this article, we are only interested in the measurement range (30–45 °C) suitable for body temperature monitoring. Another vital performance parameter, response time, is generally defined as the time consumed by the temperature sensor from applying temperature stimulation to generating a stable signal output. Some documents also define the temperature sensor's response time by the time the sensor temperature rises from \(T_{{{\text{sensor}}}}\) to 90% of the temperature rise (\(T_{0}\)) [145]. It is related to the thermal response of the active material itself and reflects the rapid response ability of the temperature sensor to temperature. In terms of applications, such as real-time human body health-monitoring products and wearable artificial intelligent elements with an instant response, all have a shorter response time.
The sensor ability to sense the smallest amount of change to be measured is defined as resolution. In other words, the input quantity starts to change from a non-zero value. At this time, if the input change value does not exceed an absolute value, and the sensor's output does not change, it means that the sensor cannot distinguish the change of the input quantity. Accuracy refers to the ratio of the value of plus or minus three standard deviations near the real value to the range, the maximum difference between the measured value and the real value, and the degree of dispersion of the measured value. For measuring instruments, accuracy is a qualitative concept and generally does not require numerical expression. Because the normal fluctuations of human body temperature within a day are small, high resolution or high accuracy is significant for flexible temperature sensors for body temperature monitoring, determining the broader application of flexible temperature sensors.
Repeatability is the degree of inconsistency of the measurement results for the same excitation quantity when the measurement system performs multiple (more than 3) measurements on the full range in the same direction under the same working conditions. In a flexible temperature sensor, durability means that it maintains a stable sensing function and a complete device capability under a long-term use environment. A flexible temperature sensor with high durability and high repeatability can meet the basic requirements of long-term stable use. Linearity usually defines as the degree of deviation between the actual input–output relationship curve and the ideal fitting curve, usually expressed as a percentage. Therefore, in the linear range, the output signal will be more accurate and reliable. High linearity is also conducive to the input–output signal calibration process and subsequent data optimization processing [58]. The demand for the development of flexible temperature sensors with high linearity is also increasing.
Applications
The ability to live organisms to respond accurately and quickly to external environmental stimuli is an essential feature of life. The induction of temperature allows humans to predict dangers and respond to diseases. Abnormal changes in body temperature often play a crucial reminder and help for early prevention [179]. With the research and development of flexible electronic devices, such as electronic skins, smart health monitoring systems, smart textiles, and biomedical equipment, sensors with the multi-functional signal acquisition are necessary [180, 181]. Among them, the flexible temperature sensor is an indispensable and vital part of medical and health applications [96]. In the past few years, the progress of new materials, new fabricating technologies, and unique sensing methods have provided an essential reference and a solid foundation for the development of a new type of skin-like flexible temperature sensor. In these sensors, some basic performance parameters such as sensitivity, resolution, and response time even exceed natural skin. Although, after a lot of basic and applied research, research results can be transformed from the laboratory to real-world use. However, there are still many challenges waiting to be solved in practical applications. In the following content, we will summarize recent flexible temperature sensors' results in flexible electronics applications with unique, excellent, and practical application examples.
Flexible sensors have the characteristics of large-area deformability, lightweight, and portability, which can realize functions that traditional sensors cannot. Electronic skin integrates various sensors and conductors on a flexible substrate to form a highly flexible and elastic sensor similar to the skin. It converts external stimuli such as pressure, temperature, humidity, and hardness into electrical signals and transmits them to the computer for processing, even can recognize regular objects [14]. An electronic skin with similar functions is a necessary feature of future robots to achieve perception in an unstructured environment. The electronic skin enables the robot to perceive changes in the external environment as sharply as a real person. Although the principle of electronic skin is simple, there are still challenges in covering the surface of the robot with electronic skin. The use of the electronic skin determines, that is, maintaining the device's integrity while maintaining the sensing performance during mechanical deformation. The ideal method of flexible electronic skin is patterned fabricating. Using solution materials, directly deposited on the substrate through printing technology to form patterns, and achieve roll-to-roll (R2R) large-area fabricating under normal temperature and pressure so that the skin has the advantages of considerable size, high yield, low production cost, and environmental protection. Someya et al. [182] have developed an electronic mesh skin with flexibility, large area, integrated temperature, and pressure-sensing capabilities, as shown in the figure. The stretchable electronic skin has multiple heats, and pressure sensors distributed at the nodes and read data through OTFT. Among them, the thermal sensor array is based on organic diodes, prepared on polyethylene naphthalate (PEN) coated with indium tin oxide (ITO) on the surface. The thermal film is mechanically cut by a numerical control cutting machine, and the R2R process prepares the network structure, and then the network film is combined by lamination to complete the preparation of the thermal sensor array. The parylene protective layer is placed on the organic semiconductor layer to act as a flexible gas barrier layer, extending the device's durability from days to weeks and avoiding mechanical damage to transistors and diodes during testing. When the sensor is in its original state, the space is square, and it becomes a rhombus after being stretched, and it can still maintain excellent electrical characteristics when stretched 25% (shown in Fig. 10a). The establishment of the mesh structure expands the use of electronic skin. The distributed structure of multiple parameters and multiple nodes also makes it possible to monitor irregularly shaped objects. A kind of epidermal electronics proposed by Kim et al. [53] refers to ultra-thin flexible electronic devices fixed on the skin's surface (in Fig. 10b). Only through van der waals force can it fit perfectly with the skin and sense the temperature, strain, and dynamic response. Potential applications include physiological state detection (electroencephalogram, electrocardiogram, electromyography), wound detection or treatment, biological/chemical perception, human–computer interaction interface, wireless communication, etcetera. Integrating all devices in the measurement device in a completely different way integrates a variety of functional sensors (such as temperature, strain, electrophysiology), micro-scale light emitting diodes (LEDs), active/passive circuit units (transistors, diodes, resistors), wireless power supply coils, wireless radio frequency communication devices (high-frequency inductors, capacitors, oscillator, and antenna). Skin electronics has the characteristics of ultra-thin, low elastic modulus, lightweight, and ductility. The device is fabricated in the form of winding-shaped filament nanowires or micro-nano thin films, enabling the system to withstand more significant elastic deformation. It can be easily transferred to the skin surface through the soft-touch process, just like a tattoo sticker. Although the electronic skin has wealthy functions, the resonance frequency will drift when the strain exceeds 12%. Besides, the durability of ultra-thin flexible devices also requires attention. The shortcomings mentioned above need to be considered when designing and fabricating future electronic skin systems.
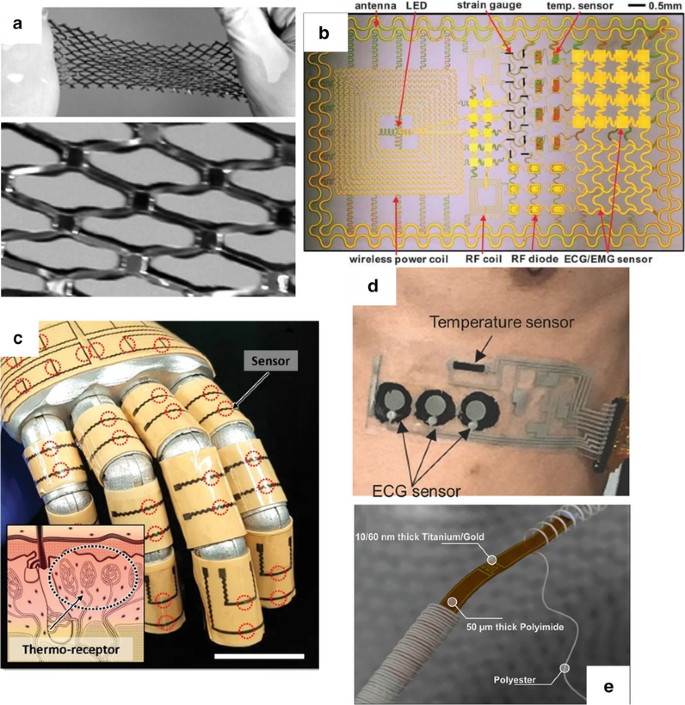
Application demonstration of flexible temperature sensor. a A plastic film with organic transistors and pressure-sensitive rubber is processed mechanically to form a unique net-shaped structure, which makes a film device extendable by 25%. A magnified view of extended net-structures is also shown [187]. b Image of a demonstration platform for multifunctional electronics with physical properties matched to the epidermis [155]. c Model hand covered with the temperature-sensitive artificial skin with enlarged illustration of skin thermos-receptors [170]. d Photo of the fabricated device [184]. e Concept of the flexible temperature sensor embedded within the fibres of a textile yarn [185]
The development of a wearable health monitoring system is for collecting human body thermal parameters:body temperature, epidermal temperature, heat flow [183], etcetera. For observation and inference such as metabolism, fever, disease infection, skin healing, thermal adaptation (implants, prostheses), etc. Compared with the limitations of traditional monitoring systems in the use cases. Flexible wearable temperature monitoring systems have demonstrated more flexible applications and excellent thermal parameter monitoring effects in recent studies—the m-LRS NiO temperature sensor system fabricated by the Shin et al. team that we introduced earlier. In an experiment to verify the potential of its system as an electronic skin application. As shown in the Fig. 10c, three side-by-side temperature sensors attached to the robot finger can make an accurate flow rate of hot or cold water flowing in the microfluidic pipe, flow direction, and temperature response. Besides, it has a high-resolution capability for the temperature increase caused by infrared heat radiation. Moreover, the high-curvature fit feature allows the temperature sensor system also to monitor breathing to provide early warning of abnormal respiratory symptoms caused by disease or poisoning. Experiments on breathing changes before and after exercise have verified the system's effectiveness in monitoring small changes in human breathing and temperature. The holistic fabricating technology they put forward provides useful help for the large-scale development of the healthcare system and electronic skin. Webb etal。 [145] reported two types of flexible, ultra-thin, and sensitive temperature sensors/actuators with different structures, namely, a TCR sensor array with a serpentine network formed by Cr/Au fabricated by microlithography technology and a TCR sensor array after deposition. Corrosion nano PIN sensor, the two sensors have high sensitivity, rapid response, and high precision. Non-invasive monitoring of the subtle changes in skin surface temperature caused by changes in human blood flow caused by external stimuli can be realized. By accurately measuring skin thermal conductivity and monitoring skin moisture, it has practical application value for health management such as blood sugar monitoring, drug delivery and absorption, and wound or malignant tumor changes. For a flexible sensor system that needs to monitor health status based on vital signals, easy fabricating, secure attachment, and strong biological adaptability are essential. As Fig. 10d shown, Yamamoto et al. [184] developed a gel-free viscous electrode that is more suitable for electrocardiogram (ECG) sensors. A temperature sensor fabricated by patterned printing is assembled to form a flexible sensor system that can monitor the occurrence of dehydration or heatstroke symptoms. The use of biocompatible materials solves the problem that traditional gels cannot be attached to the human body for a long time. The open, flexible sensing system platform can also add various sensors to develop a broader range of applications. The temperature and heat transfer properties of the skin can be characterized as critical information for clinical medicine and basic research on skin physiology. In terms of thermal adaptation, it is crucial to monitor and predict the residual limb's skin temperature. For example, the prosthesis gaps create a warm and humid micro-environment, which promotes the growth of bacteria and causes inflammation of the skin. Local skin temperature monitoring uses flexible temperature sensors hidden in daily textiles. Due to its high consistency, it is very suitable for non-existent monitoring of skin temperature, which significantly benefits patients and medical staff. Lugoda et al. [185] used different industrial yarn fabricating processes to integrate flexible temperature sensors into fabrics, and studied the sensing effect of embedding flexible temperature sensors in textile yarns. The bending resistance and repeatability of the temperature sensor embedded in the yarn are verified. The elongated sensor is made by patterning a Ti/Au layer deposited on a PI film (see in Fig. 10e). Experiments with the temperature sensor embedded in the armband have verified that this sensor yarn can be used to fabricate intelligent temperature-measuring textile garments. Smart temperature measuring textiles can be used for thermal adaptation monitoring applications such as the detection of thermal discomfort in prostheses, socks for early prediction of diabetic foot ulcers [186], textiles that continuously measure the body temperature of babies, and bandages for monitoring wound infections. The use of industrial yarn fabricating technology to integrate flexible temperature sensors makes large-scale smart textile fabricating possible.
With the development of flexible electronics, the application of multifunctional flexible wearable devices in health care, smart monitoring equipment, human–computer interaction/combination, smart robots, and other related fields have become more and more extensive. Flexible temperature sensors have strong practicability in flexible wearable electronic devices, especially for monitoring human health, which is inseparable from accurate temperature control. The introduction of the wireless transmission function allows people to analyze and utilize the temperature sensor's measurement results. Honda et al. [133] proposed a wearable human–computer interaction device, a patterned "smart bandage" with drug delivery function. The device uses low-cost patterned printing technology to integrate temperature sensors, MEMS-structured capacitive tactile sensors, and wireless coils on a flexible substrate. Figure 11a shows the physical object of the flexible device on the arm. A drug delivery pump (DDP) made by soft lithography and a microchannel for drug discharge can be added to supply drugs to wounds or internal parts to improve the wearer's health. As a wearable interactive humanized health monitoring wireless device application, it will play a positive role in future wearable electronic applications. Intelligent wound patch:wounds after trauma are infected and difficult to heal. Under traditional wound dressings, the healing process of wounds is often unpredictable, and satisfactory repair results cannot be obtained. In wound infection or healing, temperature changes different from the normal body temperature will occur. Therefore, monitoring the subtle temperature changes at the wound is quite necessary for ideal wound healing [188]. To eliminate the "black box" state in the wound healing process and grasp the wound's situation in real-time, Lou et al. [189] proposed a transparent and soft, closed-loop wound healing system to promote wound healing real-time monitoring of the wound. The flexible wound healing system (FWHS) and flexible wound temperature sensing device (FWTSD) likes the form of band-aids, their flexible device in the shape of a double-layered band-aid can be directly attached to the wound. The upper layer is a flexible temperature sensing layer composed of temperature sensors, power management circuits, and data processing circuits. The lower layer is a collagen-chitosan dermis equivalent for skin regeneration. A customized software application (app) installed on the smartphone receives data from the sensing layer, displaying and analyzing wound temperature in real-time (Fig. 11b). The system has high sensitivity and stability, good ductility, reliability, and biocompatibility. In addition to monitoring the normal wound regeneration process, in the biological experiments, timely warning of severe wound infection was achieved. A smart wound healing system with growth promotion, real-time temperature monitoring, wireless transmission, and visualization that integrates wound monitoring, early warning, and on-demand intervention may occur in the future. If the wound after an injury is severely infected and is not treated in time, more serious damage to the body may occur. Timely monitoring of wound conditions and rapid, effective diagnosis and treatment are urgent needs to reduce the occurrence and aggravation of wound infection complications. In another study, Pang et al. [190] proposed a flexible smart bandage with a double-layer structure with high sensitivity, good durability, and remote control. The upper layer is packaged in PDMS connected and integrated through a serpentine wire. The temperature sensor, ultraviolet (UV) light-emitting diode, power supply, and signal processing circuit are designed, and the lower layer is designed with a UV-responsive antibacterial hydrogel. When the temperature sensor detects an abnormal temperature exceeding the threshold, it will wirelessly transmit the signal to the smart device. Moreover, control the in-situ ultraviolet radiation through the terminal application to release antibiotics from the underlying hydrogel and apply it to the wound to achieve early infection diagnosis and processing. The system can monitor wound status in real-time, diagnose accurately, and treat on-demand. The research of this intelligent trauma diagnosis system provides new strategies and diagnostic and treatment ideas for the prevention and treatment of traumatic diseases such as wound treatment and diabetic ulcers. The popularization and application of such systems also have significant prospects. The development of advanced surgical tools is an important measure to improve human health. The advancement of flexible electronics provides reliable support for the flexibility and miniaturization of clinical medical instruments. Simultaneously, the function of flexible devices is enriched, allowing clinical instruments to perform multiple operations on diseased parts in a short period, reducing the risk of surgery. In Fig. 11c,d, Kim et al. [191] invented a direct integration of a multifunctional element group, including a flexible temperature sensor array with the elastic film of a traditional balloon catheter, which provides a variety of functions for clinical applications. The use of this balloon catheter in live animal experiments verified the instrument's ability to provide key information about the depth of the lesion, contact pressure, blood flow or local temperature, and the radiofrequency electrodes on the balloon for tissue controlled local ablation. Specific use in cardiac ablation therapy. In another study, they developed two thin and bendable flexible temperature sensor applications. Used for medical sutures, one of the simplest and most widely used devices in clinical medicine. One is an ultra-thin suture line based on the integrated Au thermal actuator and silicon nanodiode temperature sensor on the biofiber membrane. The other suture line is two \(1 \times 4\) Pt metal temperature sensor arrays formed between Au wires on both sides of the substrate. To achieve the purpose of helping biological wounds heal and monitoring wound recovery. It is full of challenges to build semiconductor devices and sensors and other components on a biologically adaptive platform that touches the flexible curved surface of the human body.
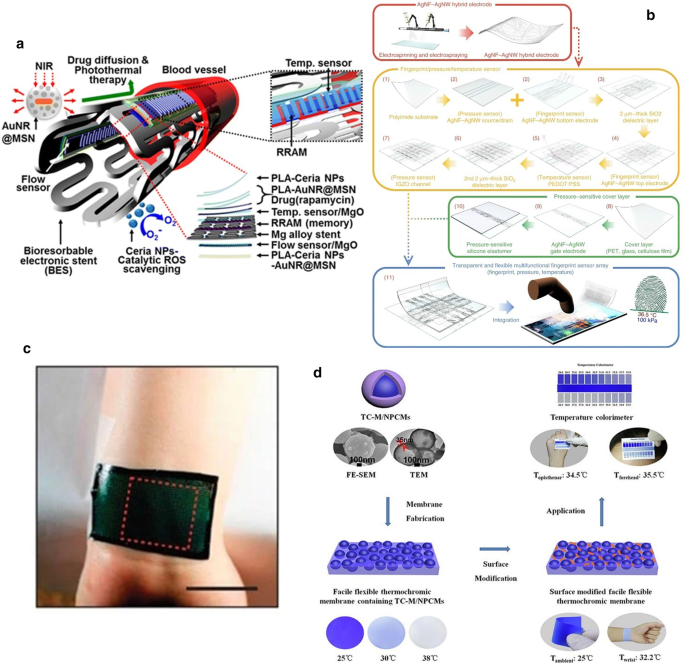
Application demonstration of flexible temperature sensor. a Photos of the smart bandage integrated with touch and temperature sensors, a wireless coil, and a DDP [133]. b The application scenario of FWHS. The FWTSD with a Band-Aid shape tightly contacts with the wound site. Temperature variation is detected by temperature sensor [189]. c Optical image of a multifunctional balloon catheter in deflated and inflated states [191]. d Optimized mechanical structure shown in a schematic illustration when wrapping [53]. e Image of bioresorbable pressure and temperature sensors integrated with dissolvable metal interconnects and wires. f Diagram of a bioresorbable sensor system in the in a rat's skull [193]
As we all know, some operations will leave objects different from the body's tissues such as steel plates, stents, pacemakers, implants, etcetera, inside the body, which will cause discomfort to the body [192]. The development of absorbable devices will optimize surgical implantation equipment Post-access problems reduce the risk of surgery and the difficulty of post-wound healing.カンら。 [193] reported a multifunctional silicon sensor. The experiment of implanting the sensor in rats' brains proved that all the materials (polylactic-glycolic acid, silicon nanomembranes (Si-NMs), nanoporous silicon, SiO2 ) constituting the sensor could be used. Naturally absorbed through hydrolysis and/or metabolism, no need to extract again. It can continuously monitor parameters such as intracranial pressure and temperature, which illustrates the performance advantages of absorbable devices for treating brain trauma. The emergence of implantable vascular stents provides strong support for the smooth progress of interventional surgery. The vascular stent can expand the blood vessel through a continuous structure in the blocked blood vessel to restore blood flow. However, the inflammation caused by traditional vascular stents in the body for a long time is still difficult to be diagnosed and cured.息子等。 [194] introduced the bioabsorbable electronic stent (BES) applications based on bioabsorbable and bioinert nanomaterials.(Fig. 11e,f) Integrated flow sensing, temperature monitoring, wireless power/data transmission, and inflammation suppression, local drug delivery, and hyperthermia device. The Mg temperature and flow sensor are composed of an adhesion layer, a fiber-shaped Mg resistance (sensing unit), and an outer encapsulation layer (MgO and polylactic acid(PLA)) (as shown in Fig. 12a). Experimental studies have shown that combining bio-absorbable electronic implants and bio-inert therapeutic nanoparticles in intravascular smart stent systems has not yet been recognized.
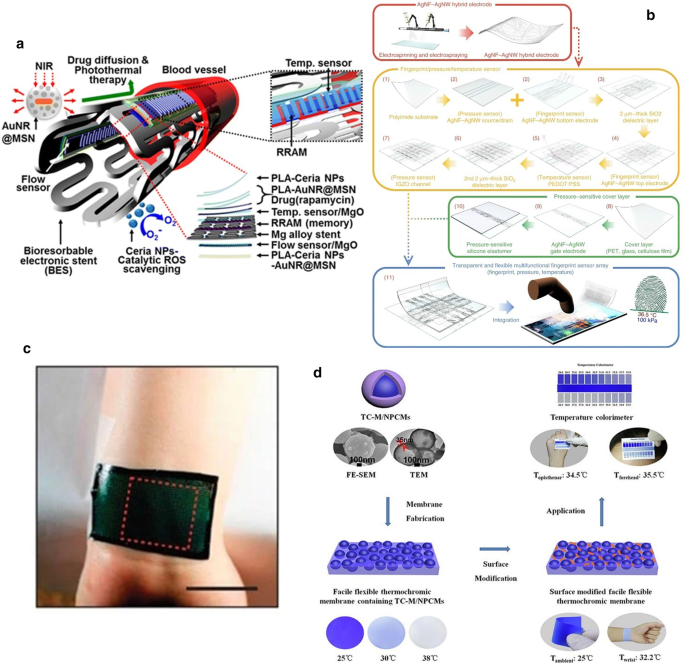
Application demonstration of flexible temperature sensor. a Schematic illustration of the BES (left), its top view (top right), and the layer information (bottom right). The BES includes bioresorbable temperature/flow sensors, memory modules and bioresorbable/bioinert therapeutic nanoparticles [194]. b The fabrication process for the multiplexed fingerprint sensor [196]. c Optical images of an e-TLC device on the wrist during an occlusion test (scale bar, 3 cm) [15]. d Facile flexible reversible thermochromic membranes based on micro/nanoencapsulated phase change materials for wearable temperature sensor [198]
Temperature visualization makes the function of flexible temperature sensors more prominent. It makes up for the disadvantages of traditional infrared imaging equipment that are expensive, unfavorable for carrying, and inaccurate in measuring dynamic objects. The appearance of high-intensity focused ultrasound (HIFU [55]), smart device monitoring, and other occasions will make temperature measurement more accurate and useful [195]. An et al. [196] developed a PEDOT:PSS temperature sensor with ultra-long silver nanofibers (AgNFs) and silver nanowires (AgNWs) hybrid network as a high-performance transparent electrode, layer, and patterned fabricating, using multiplexing technology to create a capacitive flexible and transparent multifunctional sensor array (Fig. 12b). The capacitance change is 17 times higher than that of a pattern sensor that also uses ITO electrodes. In the future, the visual display of fingerprints, pressure, and temperature changes between the fingers on the flexible sensor can be realized simultaneously on smart devices. Applying in the fingerprint recognition function, the security function of smart mobile devices can improve more security. Gao etal。 [15] introduced a light-emitting device that combines a colorimetric temperature indicator with wireless, patterned, and stretchable flexible electronic technology. A large-scale thermochromic liquid crystal (TLC) pixel array is formed on a thin elastic substrate in combination with colorimetric reading and radio frequency (RF) drive to map the thermal characteristics of the skin. Under the premise of non-invasiveness, the sensor system uses radio frequency signals to control local heating to survey and map skin temperature with high-temperature accuracy (50 mK) and high spatial resolution. The blood flow under the skin is evaluated by the reactive hyperemia test (see in Fig. 12c). Also, hydration analysis reflects skin health problems. The figure verifies that the device can quickly visually respond to small changes in blood flow. Reflect cardiovascular and skin health problems through hydration analysis. Like the fluoroscopy capability of an infrared camera, the device can be used for core temperature and wound healing monitoring, near-body implant device inspection, malignant tumor cancer screening, and other biomedical-related functions. The unique feature is that the flexible device can be read using a mobile phone, and it can be worn for a long time. The temperature visualization system provides a massive prospect for the description of the thermal properties of the skin. It makes efforts to provide useful indicators for determining the human health and physiological state. In another study, Kim et al. [197] provided a feasible idea using thermochromic materials to observe temperature changes directly. The difference is that their description of the temperature change does not have a specific numerical display, and can only judge the approximate temperature change range. However, as shown in Fig. 12d, He et al. optimized the accuracy of the mapping temperature of thermochromic materials, which can reflect the specific value of the temperature more accurately [198]. In future research, the development of thermochromic materials sensitive to temperature and can accurately respond to temperature will also gradually becoming one research focus. In particular, the reverse cushioning material they made shown a color similar to human skin. Besides, they used 3D printing technology to fabricate the flexible pressure sensor, simplifying the fabricating process, because the sensor structure parameters can be adjusted, showing customizable functions, and achieving the purpose of overall structural fabricating. In the future, 3D printing is the best candidate for the development of unique functional structures [199]. Because this technology can achieve the overall fabricating of the device, it simplifies the fabricating process and ensures integrated molding requirements. It will also gain enormous popularity in the field of flexible sensors.
結論
This article reviews the recent research progress of high-sensitivity flexible temperature sensors in human body temperature monitoring, heat-sensitive materials, fabricating strategies, basic performance, and applications. As a relatively stable dynamic variable in the human body, body temperature or local temperature (trauma) may have different degrees of small fluctuations (about 0.5 °C) under the influence of emotions or physiological activities. The monitoring temperature of the flexible temperature sensor needs to be comparable. Traditional infrared cameras have smaller temperature resolution (< 0.1 °C). Besides, timely and fast monitoring of body temperature is another key to breakthrough. The current temperature sensor response time can be within a few milliseconds, but there is a problem of too long reset time. How to shorten the time difference between response time and reset time will directly affect sensor monitoring's efficiency and capability. With the development of multifunctional sensors, how to avoid mutual interference between multiple signals so that the stimuli of the respective responses between the signals can independently respond without mutual interference and accurately output, which has become an urgent problem to be solved and perfected for improving sensor performance. The development of flexible temperature sensors shows us a foreseeable future. In the future, flexible temperature sensors will also achieve large-area low-cost fabricating, high sensitivity, self-supply, visualization, self-healing, biodegradability, and wireless remote sensing transmission [200]. Furthermore, other functions are integrated and put into use. Sorting the collected temperature data into the health big data platform can provide the best help and data support for human future medical diagnosis. Also, patterned micro-nano fabricating technology is a good suggestion for low-cost mass production of sensors. Based on the relatively mature process flow of the printing process, the realization of integrated multifunctional large-area flexible devices is just around the corner. The current flexible temperature sensor can achieve higher sensitivity, but some sensors do not eliminate environmental factors' interference on the temperature sensor. In the future, the flexible temperature sensor used for body temperature monitoring can make efforts to combat environmental influences. Although the flexible sensor itself can be fragile and light, it needs to be connected to the power supply circuit and the power supply, which dramatically reduces the overall flexibility. In the future, for flexible temperature sensors that monitor body temperature, with the further optimization of signal acquisition methods, real-time visual data wireless transmission can be realized under more efficient self-powered conditions, which will be a vast improvement for intelligent monitoring systems. The monitoring of body surface temperature is greatly affected by the environment, while the core temperature is relatively stable. The flexible temperature sensor used for body temperature monitoring can be attached to the body surface (forehead, arm, armpit, etcetera.) to monitor the body surface temperature and even fluctuate. The core temperature with a small range can also be measured. Non-implantable flexible sensors require more improvements in wearability, biocompatibility, and durability to meet the needs of a broader range of people and become a flexible application device available to everyone. For intrusive flexible temperature sensors, whether in the process of intrusion or during the use of the sensor, minimizing damage to the body is the primary consideration. Therefore, exploring and developing biocompatible or biodegradable sensing materials and sensors is undoubtedly an improvement direction. There will be no rejection or allergic reactions in the body due to foreign bodies. In the future, flexible temperature sensors will appear on many occasions around us. The exploration of the development of flexible temperature sensors with high performance, easy fabricating, low cost, and wide application range will continue.
データと資料の可用性
該当なし。
略語
- PDMS:
-
ポリジメチルシロキサン
- PI:
-
Polyimide
- PU:
-
Polyurethane
- PET:
-
ポリエチレンテレフタレート
- PVA:
-
ポリビニルアルコール
- PVB:
-
Polyvinyl butyral
- CTE:
-
Coefficient of thermal expansion
- CB:
-
Carbon black
- CNT:
-
カーボンナノチューブ
- TCR:
-
Temperature coefficient of resistance
- EG:
-
Expanded graphite
- Gr:
-
Graphite
- PEO:
-
ポリエチレンオキシド
- PVDF:
-
ポリフッ化ビニリデン
- PEDOT:
-
PSS:Poly(3,4-ethylene dioxythiophene)-poly(styrene sulfonate)
- MWCNT:
-
多層カーボンナノチューブ
- SWCNTs:
-
Single-walled carbon nanotubes
- GO:
-
酸化グラフェン
- rGO:
-
還元型酸化グラフェン
- GNWs:
-
グラフェン
- PECVD:
-
プラズマ化学気相成長法
- TS:
-
Transparent and stretchable
- Au:
-
Gold
- Ag:
-
シルバー
- Cu:
-
銅
- Pt:
-
Platinum
- Ni:
-
ニッケル
- Al:
-
アルミニウム
- MEMS:
-
Micro-electro-mechanical system
- Cr:
-
Chromium
- OTFT:
-
Organic thin-film transistor
- NP:
-
ナノ粒子
- PE:
-
Polyethylene
- PTC:
-
Positive temperature coefficient
- VO2 :
-
Vanadium dioxide
- PAD:
-
Polymer-assisted deposition
- NiO:
-
Nickel oxide
- P3HT:
-
Poly(3-hexyl thiophene)
- PPy:
-
ポリピロール
- pNIPAM:
-
Poly (N-isopropyl acrylamide)
- SEBS:
-
Polystyrene- block-poly(ethylene-ran-butylene)-block-polystyrene
- PDPPFT4:
-
Poly(diketopyrrolopyrrole-[3,2-b]thieno[2′,3′:4,5]thieno[2,3-d]thiophene])
- PII2T:
-
Poly(isoindigo-bithiophene)
- OSCs:
-
Organic semiconductors
- TENG:
-
Thermoelectric nanogenerator
- DN:
-
Double-network
- DMSO:
-
ジメチルスルホキシド
- P (VDF-TrFE):
-
Copolymer polyvinylidene fluoride
- IR:
-
赤外線
- FET:
-
電界効果トランジスタ
- BT:
-
BaTiO 3
- ZnO:
-
酸化亜鉛
- MFSOTE:
-
Microstructure-frame-supported organic thermoelectric
- PVD:
-
物理蒸着
- CVD:
-
化学蒸着
- AlPcCl:
-
Aluminum phthalocyanine chloride
- RF-PECVD:
-
Radio frequency plasma enhanced chemical vapor deposition
- FCCVD:
-
Floating catalyst chemical vapor deposition
- PEI:
-
Polyetherimide
- LS:
-
Laser writing
- R2R:
-
Roll-to-roll
- EES:
-
Electronic skin system
- LDW:
-
Laser direct writing
- GNR:
-
Graphene nanoribbon
- tascPLA:
-
Three-arm three-dimensional composite polylactide, polylactic acid
- XRD:
-
X線回折
- m-LRS:
-
Laser-induced reduction sintering
- NTC:
-
Negative temperature coefficient
- ITO:
-
インジウムスズ酸化物
- LED:
-
Light emitting diodes
- ECG:
-
心電図
- DDP:
-
Drug delivery pump
- FWHS:
-
Flexible wound healing system
- FWTSD:
-
Flexible wound temperature sensing device
- UV:
-
紫外線
- Si-NMs:
-
Silicon nanomembranes
- BES:
-
Bioabsorbable electronic stent
- HIFU:
-
High-intensity focused ultrasound
- AgNFs:
-
Silver nanofibers
- AgNWs:
-
Silver nanowires
- TLC:
-
Thermochromic liquid crystal
- RF:
-
無線周波数
ナノマテリアル