ナノ粒子の毒性の物理的および化学的性質への依存性
要約
ナノ粒子(NP)合成の方法に関する研究、それらの特性の分析、およびそれらの応用の新しい分野の探求は、現代のナノテクノロジーの最前線にあります。水溶性NPを操作する可能性は、さまざまな基礎的および応用的な生物医学研究での使用への道を開きました。現在、NPは、遺伝性および自己免疫疾患、悪性腫瘍、およびその他の多くの障害の多数の分子マーカーの画像診断に使用されています。 NPは、薬物の放出と蓄積の制御可能なパラメーターを使用して、組織や臓器への薬物の標的化送達にも使用されます。さらに、有効成分としてのNPの使用例があります。たとえば、光線力学療法やNPの取り込みと加熱による温熱腫瘍破壊における光増感剤などです。ただし、生物に対するNPの高い毒性は、invivoでの使用を妨げる強力な制限要因です。 NPの毒性作用に関する現在の研究は、それらの有害作用の標的とメカニズムを特定することを目的として、細胞培養モデルで実施されています。動物モデルにおけるNP輸送、蓄積、分解、および除去のパターンに関する研究。このレビューは、生体系に対するNP毒性のメカニズムがそれらの物理的および化学的特性にどのように関連しているかについて、利用可能なデータを体系化して要約します。
背景
国際標準化機構は、ナノ粒子(NP)を、1次元、2次元、または3次元のサイズが1〜100nmの範囲内にある構造として定義しています。サイズとは別に、NPは物理的パラメータ(電荷など)の観点から分類できます。 NPコアまたはシェルの組成などの化学的特性。形状(チューブ、フィルム、ロッドなど);起源:このレビューの焦点である天然のNP(火山灰、ウイルス粒子などに含まれるNP)と人工のNP。
ナノ粒子は、電子工学、農業、繊維生産、医学、および他の多くの産業や科学で広く使用されるようになりました。しかし、生物に対するNP毒性は、病気の治療と診断におけるそれらの使用を制限する主な要因です。現在、研究者はしばしば、NPの正の治療効果とそれらの毒性に関連する副作用との間のバランスの問題に直面しています。この点で、invitro(細胞株)とinvivo(実験動物)の間の毒性を推定するための適切な実験モデルの選択が最も重要です。個々の細胞成分および個々の組織に対するNP毒性効果は、in vitroモデルで分析するのが簡単ですが、in vivo実験では、個々の臓器または体全体のNP毒性を推定することができます。さらに、NPの考えられる毒性効果は、NPの濃度、生物との相互作用の持続時間、体液中での安定性、および組織や臓器への蓄積能力によって異なります。人間の病気の診断と治療に使用できる安全で生体適合性のあるNPの開発は、NP毒性の根底にあるすべての要因とメカニズムの間の相互作用の完全な理解にのみ基づくことができます。
ナノ粒子の医療用途
医学では、NPは診断または治療目的で使用できます。診断では、生体分子や病原体を検出するための蛍光標識として、また磁気共鳴やその他の研究で造影剤として機能します。さらに、NPは、タンパク質やポリヌクレオチド物質を含む薬物の標的化送達に使用できます。光線力学療法と腫瘍の熱破壊、および補綴修復[1,2,3,4,5,6]。いくつかのタイプのNPは、すでにクリニックでドラッグデリバリーや腫瘍細胞イメージングに使用されています[7,8,9]。
ゴールドNPの使用例は最近蓄積されています。それらは化学療法剤および他の薬物の効率的な担体であることが証明されています。ゴールドNPは生体適合性が高いです。しかし、物質としての金は生物学的対象に対して不活性ですが、遅延毒性効果がないことに関する決定的なデータがまだないため、金NPについても同じことが言えるとは言えません[10]。金のNPに加えて、ミセル、リポソーム[11]、および「捕捉分子」が付着したポリマー[12]に基づくものは、すでに薬物担体として使用されています。単層および多層ナノチューブは、ドラッグデリバリーに使用されるNPの良い例です。それらは、標的化送達のためにさまざまな官能基および分子を結合するのに適しており、それらの独特の形状により、生物学的障壁を選択的に貫通することができます[13]。薬物の媒体としてのNPの使用は、送達の特異性を高め、治療効果を達成および維持するために必要なNPの最小量を減らし、それによって最終的な毒性を低減します。これは、毒性が高く短命の化学療法剤および放射線療法剤の場合に特に重要です[14]。
量子ドット(QD)は、臨床使用の可能性が高いNPの別のグループを構成します。 QDは、サイズが2〜10nmの半導体ナノ結晶です。赤外線領域を含むさまざまなスペクトル領域での蛍光能力[15]により、細胞、細胞構造、または病原性生物剤のラベル付けとイメージング、および細胞、組織、体全体のさまざまなプロセスに適しています[15]。 16,17,18]、これは重要な診断上の意味を持っています[19、20]。超常磁性酸化鉄に基づくNPは、肝臓、骨髄、およびリンパ節組織を画像化するための磁気共鳴断層撮影法(MRT)の造影剤として効率的に使用されます[21]。リン脂質で機能化された放射性標識単層カーボンナノチューブを使用して、インテグリン含有腫瘍を標識し、その後、マウスでの実験で陽電子放出断層撮影法を用いて検出した例もあります[22]。
ナノ粒子は、グルコースレベルの測定[23]、特定のDNAフラグメントと領域の検出[24]、細菌細胞の識別[25]のためのカーボンナノチューブに基づくものなど、バイオセンサーの設計にも使用されています。
銀(または銀含有)NPは、抗菌効果と細胞増殖抑制効果を発揮します。このため、それらは、包帯、手術器具、プロテーゼ、および避妊薬の治療など、医学で広く使用されています[13、22]。シルバーNPは、化粧品業界で効果的かつ安全な保存剤として機能することが報告されています[26]。
ただし、NPの化学成分の多くを医学で使用することの安全性が証明されたとしても、NPは依然として非常に毒性が高い可能性があります。毒性作用は、生体システムとの相互作用の特定のメカニズムの根底にあるそれらの独特の物理的および化学的特性によって引き起こされる可能性があります。一般に、これは、NPの潜在的な毒性作用の原因とメカニズムを研究することの重要性を決定します。
ナノ粒子毒性のメカニズム
NPの毒性は、主に、サイズ、形状、比表面積、表面電荷、触媒活性、表面上のシェルと活性基の有無など、物理的および化学的特性によって決まります。
NPのサイズが小さいため、上皮や内皮のバリアを通過してリンパ液や血液に浸透し、血流やリンパ流によって脳、心臓、肝臓、腎臓、脾臓、骨髄などのさまざまな臓器や組織に運ばれます。神経系[27、28]であり、トランスサイトーシスメカニズムによって細胞内に輸送されるか、細胞膜を介して細胞内に拡散するだけです。ナノマテリアルはまた、摂取を通じて血流へのアクセスを増加させる可能性があります[29、30]。一部のナノマテリアルは皮膚に浸透する可能性があり[31、32]、さらに大きな微粒子は屈曲すると皮膚に浸透する可能性があります[33]。ナノ粒子は、サイズが小さいため、炎症部位の内皮、上皮(腸管や肝臓など)、腫瘍を介して溢出するか、微小毛細血管に浸透する可能性があります[34]。 NPの身体への毒性作用をモデル化した実験では、NPが血小板凝集[35]、上下呼吸管の炎症、神経変性障害、脳卒中、心筋梗塞、およびその他の障害[36,37,38]を増強することによって血栓症を引き起こすことが示されています。 ]。 NPは、臓器、組織、細胞だけでなく、ミトコンドリアや核などの細胞小器官にも侵入する可能性があることに注意してください。これは細胞代謝を劇的に変化させ、DNA損傷、突然変異、細胞死を引き起こす可能性があります[39]。
QDの毒性は、環境因子による酸化の際に、カドミウム、鉛、ヒ素などのコアに含まれる金属の遊離イオンの漏れに直接関係していることが示されています。 QDはミトコンドリアに吸収され、細胞小器官の形態学的変化や機能障害を引き起こす可能性があります[40]。カドミウムベースのQDの細胞への侵入と遊離Cd 2+ の形成 イオンは酸化ストレスを引き起こします[41、42]。
最近の研究によると、肺組織が約50 nmのサイズのNPと接触すると、I型肺胞細胞の膜が穿孔され、その結果、NPが細胞に侵入することが示されています。これは、乳酸デヒドロゲナーゼの放出によって証明されるように、次に、細胞壊死を引き起こします[43]。 QDの浸透が細胞膜の流動性を高めるという証拠があります[44]。一方、膜脂質の過酸化によって誘発される活性酸素種(ROS)の形成は、膜の柔軟性の喪失につながる可能性があり、異常に高い流動性と同様に、必然的に細胞死を引き起こします。
NPと細胞骨格との相互作用も細胞骨格に損傷を与える可能性があります。たとえば、TiO 2 NPはチューブリンのコンフォメーション変化を誘発し、その重合を阻害します[45]。これは細胞内輸送、細胞分裂、および細胞移動を妨害します。ヒト臍帯静脈内皮細胞(HUVEC)では、細胞骨格の損傷により、細胞骨格を細胞外マトリックスに結合する配位接着複合体の成熟が妨げられ、それによって血管網の形成が妨げられます[46]。
さらに、NP細胞毒性は、細胞分化およびタンパク質合成を妨害するだけでなく、炎症誘発性遺伝子および炎症性メディエーターの合成を活性化する可能性があります。通常の保護メカニズムはNPに影響を与えないことに特に注意する必要があります。大きなPEG化ナノ粒子のマクロファージ取り込みは、小さなナノ粒子の取り込みよりも効率的であり、体内にNPが蓄積します[47]。超常磁性酸化鉄NPは、幹細胞の骨形成分化を妨害または完全に抑制し、シグナル分子、腫瘍抗原などの合成を活性化することが実証されています[48、49]。さらに、NPと細胞との相互作用は、リソソームの形成に関与する遺伝子の発現を増強し[50]、それらの機能を妨害し[51]、タンパク質合成を阻害します[52、53]。肺上皮細胞およびヒト腫瘍細胞株に対する異なる組成のNPの毒性作用に関する研究は、NPが炎症メディエーター、例えばインターロイキン8の合成を刺激することを示しています[54]。 invitroおよびinvivoで炎症性サイトカインの発現を研究したParkによると、インターロイキン1ベータ(IL-1β)および腫瘍壊死因子アルファ(TNFα)の発現は、シリコンNPに応答して増強されます[55]。
>酸化、およびNPのシェルと表面に対するさまざまな酵素の作用により、NPの分解とフリーラジカルの放出が起こります。酵素の酸化と不活性化、突然変異誘発、および細胞死につながる化学反応の妨害として表されるフリーラジカルの毒性効果に加えて、NPの分解はそれら自身の機能の変化または喪失につながります(例えば、磁気モーメントの喪失)および蛍光スペクトルおよび輸送または他の機能の変化)[56、57]。
要約すると、NP細胞毒性の最も一般的なメカニズムは次のとおりです。
- 1。
NPは、ROSやその他のフリーラジカルの形成を介して酸化を引き起こす可能性があります。
- 2。
NPは、細胞膜に穴を開けることによって細胞膜に損傷を与える可能性があります。
- 3。
NPは細胞骨格の構成要素に損傷を与え、細胞内輸送と細胞分裂を妨害します。
- 4。
NPは転写を妨害し、DNAに損傷を与えるため、突然変異誘発を促進します。
- 5。
NPはミトコンドリアに損傷を与え、その代謝を妨害します。これにより、細胞のエネルギーの不均衡が生じます。
- 6。
NPはリソソームの形成を妨害し、それによってオートファジーと高分子の分解を妨げ、アポトーシスを引き起こします。
- 7。
NPは、膜タンパク質の構造変化を引き起こし、細胞間輸送を含む、細胞内外への物質の輸送を妨害します。
- 8。
NPは、細胞代謝の正常なメカニズム、および組織や臓器の代謝を妨害することにより、炎症性メディエーターの合成を活性化します(図1)。
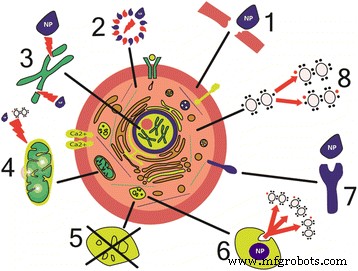
ナノ粒子による細胞損傷のメカニズム。 (1)膜の物理的損傷[43、67、75]。 (2)細胞骨格成分の構造変化[45、46]。 (3)DNAの転写および酸化的損傷の妨害[61、62]。 (4)ミトコンドリアの損傷[39、40]。 (5)リソソーム機能の障害[51]。 (6)活性酸素種の生成[61]。 (7)膜タンパク質機能の障害[172]。 (8)炎症性因子とメディエーターの合成[54、55]
NP毒性には多くのメカニズムがありますが、NPの特定の毒性効果の種類とメカニズムを、その物理的および化学的特性に応じて決定および分類する必要があります。
ナノ粒子の毒性とその物理的および化学的特性との関係
NPの毒性は、サイズ、形状、表面電荷、コアとシェルの化学組成、安定性など、物理的および化学的特性に依存すると考えられています。特に、Ohらは、1741年の細胞生存率に関連するデータサンプルを説明する307の論文のデータメタアナリシスを使用して、最近CdSe量子ドットの毒性を分析しました。 QDナノ毒性は、それらの表面特性(シェル、リガンド、および表面修飾を含む)、直径、使用される毒性アッセイの種類、および曝露時間と密接に関連していることが示されています[58]。これらの要因のどれが最も重要であるかは、特定の実験タスクとモデルによって決定されます。したがって、ここでは各要素を個別に検討します。
ナノ粒子のサイズと毒性
NPのサイズと表面積は重要な役割を果たし、主にNPと生体系との相互作用の独自のメカニズムを決定します。 NPは、比表面積が非常に大きいという特徴があり、これが高い反応能力と触媒活性を決定します。 NPのサイズ(1〜100 nm)は、タンパク質小球のサイズ(2〜10 nm)、DNAらせんの直径(2 nm)、細胞膜の厚さ(10 nm)に匹敵するため、簡単に処理できます。細胞と細胞小器官を入力します。たとえば、Huo etal。は、6 nm以下の金のNPが細胞核に効果的に侵入するのに対し、大きなNP(10または16 nm)は細胞膜を透過するだけで、細胞質にのみ見られることを示しています。これは、サイズが数ナノメートルのNPは、核に入ることができない10nm以上のNPよりも毒性が高いことを意味します[59]。パンら。 0.8〜15 nmの範囲で、金NPの毒性のサイズへの依存性を追跡しました。サイズが15nmのNPは、線維芽細胞、上皮細胞、マクロファージ、および黒色腫細胞に対して、1.4 nmNPよりも60倍毒性が低いことがわかっています。また、1.4 nm NPは細胞壊死を引き起こしますが(細胞培養培地に添加してから12時間以内)、1.2 nmNPは主にアポトーシスを引き起こします[60]。これらのデータは、NPが核に入る可能性があるだけでなく、NPの幾何学的サイズ(1.4 nm)とDNAの主溝の幾何学的サイズの対応により、負に帯電した糖リン酸DNAバックボーンと効果的に相互作用できることを示唆しています。転写をブロックする[61、62]。
さらに、NPのサイズは、NPが細胞や体の輸送および防御システムとどのように相互作用するかを大きく左右します。この相互作用は、順番に、体内でのそれらの分布と蓄積の動力学に影響を与えます。 [63]によるレビューペーパーは、理論的考察と多数の実験データの両方を示しており、5 nm未満のNPは通常、転座などを介して非特異的に細胞障壁を克服しますが、大きな粒子は食作用、マクロ飲作用、および特異的および非特異的輸送メカニズムによって細胞に侵入します。約25nmのNPサイズは飲作用に最適であると考えられていますが、これは細胞のサイズとタイプにも強く依存します[63、64]。インビボ実験では、静脈内投与時に10 nm未満のNPがすべての臓器および組織に急速に分布するのに対し、ほとんどの大きいNP(50〜250 nm)は肝臓、脾臓、および血液に見られることが示されています[65]。これは、大きなNPが体の特定の防御システムによって認識され、単核食細胞のシステムによって吸収されて、他の組織に入るのを防ぐことを示唆しています。さらに、Talamini etal。 NPのサイズと形状は、フィルター器官での金NPの蓄積と排泄の動態に影響を及ぼし、星のような金NPのみが肺に蓄積できると主張しました。彼らはまた、NP形状の変化が血液脳関門のNP通過を改善しないことを示しました[66]。
大きな比表面積により、細胞表面へのNPの効果的な吸着が保証されます。これは、ヒト赤血球に対する100〜600nmのメソポーラスシリコン粒子の溶血活性に関する研究で示されました[67]。サイズ100nmの粒子は、細胞破壊や細胞の形態変化を引き起こすことなく赤血球表面に効果的に吸着されましたが、600 nm粒子は膜を変形させて細胞に入り、赤血球破壊(溶血)を引き起こしました[67]。
ナノ粒子の形状と毒性
NPの特徴的な形状は、球、楕円体、円柱、シート、立方体、および棒です。 NPの毒性はそれらの形状に強く依存します。これは、さまざまな形状と化学組成の多数のNPで示されています[68、69、70、71]。たとえば、球状のNPは、ナノチューブやナノファイバーよりもエンドサイトーシスを起こしやすい[72]。単層カーボンナノチューブは、球状フラーレンと比較してカルシウムチャネルをより効果的に遮断することがわかっています[73]。
培養されたBEAS-2B細胞に対する異なる形状(針状、板状、棒状、および球状)のヒドロキシアパタイトNPの効果の比較は、板状および針状のNPがより多くの割合の死を引き起こすことを示しています。球状および棒状のNPよりも細胞[74]。これは、直接接触すると細胞や組織に損傷を与える板状および針状のNPの能力によって部分的に説明されます。 Hu etal。 [75]は、酸化グラフェンナノシートによる哺乳類細胞の損傷を研究するときに興味深いデータを取得しました。これらのNPの毒性は、細胞膜を物理的に損傷することを可能にする形状によって決定されました。しかしながら、それらの毒性は、培地中のウシ胎児血清濃度の増加とともに減少することが見出された。これは、タンパク質分子を吸着するための酸化グラフェンNPの高容量によって説明されました。これは、NP表面を覆い、それによってNPの形状を変化させ、細胞膜の損傷を部分的に防止します[75]。
ナノ粒子の化学組成と毒性
NPの毒性はそのサイズと形状に強く依存しますが、NPの化学組成や結晶構造などの他の要因の影響を無視してはなりません。 20 nmの二酸化ケイ素(SiO 2 )およびマウス線維芽細胞上の酸化亜鉛(ZnO)NPは、毒性のメカニズムが異なることを示しています。 ZnO NPは酸化ストレスを引き起こしますが、SiO 2 NPはDNA構造を変化させます[76]。
NPの毒性は、実際、主にその化学組成によって決定されます。 NPの劣化が発生する可能性があり、その程度は環境条件、たとえばpHやイオン強度に依存することが示されています。細胞と相互作用するNPの毒性作用の最も一般的な原因は、NPコアからの金属イオンの漏出です。毒性は、NPのコアの組成にも依存します。 AgやCdなどの一部の金属イオンは実際には毒性があるため、細胞に損傷を与えます。 FeやZnなどの他の金属イオンは生物学的に有用ですが、高濃度では、細胞経路を損傷する可能性があるため、高い毒性を引き起こす可能性があります。ただし、この効果は、たとえば、NPコアを短いリガンドの代わりに厚いポリマーシェル、シリカ層、または金シェルでコーティングするか、NP合成に無毒の化合物を使用することによって減らすことができます。一方、コアの組成は、他の金属の添加によって変更される可能性があります。これにより、NPの分解や体内への金属イオンの漏出に対する化学的安定性が向上する可能性があります[77]。
NPの毒性は、その結晶構造にも依存します。結晶構造と毒性の関係は、ヒトの気管支上皮細胞株と、さまざまな種類の結晶格子を持つ酸化チタンNPを使用して研究されています。ルチルのような結晶構造を持つNP(プリズム型のTiO 2 結晶)は、DNAの酸化的損傷、脂質過酸化、および小核の形成を引き起こします。これは、有糸分裂中の異常な染色体分離を示しますが、アナターゼ様の結晶構造を持つNP(八面体TiO 2 同じサイズの結晶)は無毒です[78]。 NP結晶構造は、例えば、水、体液、または他の分散媒体との相互作用の際に、環境に応じて変化する可能性があることに留意されたい。 ZnS NPの結晶格子は、水と接触するとより規則正しい構造に再配列されるという証拠があります[79]。
ナノ粒子の表面電荷と毒性
NPの表面電荷は、NPと生物学的システムとの相互作用を大きく左右するため、毒性において重要な役割を果たします[80、81]。
NP表面とその電荷は、異なる電荷を持つポリマーをグラフトすることで変更できます。 PEG(ポリエチレングリコール)または葉酸は、NPの細胞内取り込みと特定の細胞を標的とする能力を改善するためによく使用されます[82]。機能性NH2またはSH基を含む生体適合性TiO2ナノ粒子の合成も報告されています[83]。メトトレキサート、ポリエチレンイミン、デキストランなどの他の物質も、NP表面とその電荷を修飾するために使用されていました[84]。
正に帯電したNPの高い毒性は、負に帯電した中性のNPとは対照的に、細胞に容易に侵入する能力によって説明されます。これは、負に帯電した細胞膜糖タンパク質と正に帯電したNPの間の静電引力によって説明されます。 HeLaおよびNIH / 3T3細胞に対する負および正に帯電したポリスチレンNPの細胞毒性効果の比較は、後者のNPがより毒性があることを示しています。これは、正に帯電したNPがより効果的に膜を透過するだけでなく、負に帯電したDNAに強く結合し、その損傷を引き起こし、その結果、細胞周期のG0 / G1期を延長するためです。負に帯電したNPは細胞周期に影響を与えません[85]。正および負に帯電した金NPについても同様の結果が得られており、正のNPは負のNPよりも大量かつ迅速に細胞に吸収され、毒性が高くなります[86]。
正に帯電したNPは、オプソニン作用、つまり、血液や体液からの抗体や補体成分などの食作用を促進するタンパク質の吸着能力が強化されています[87]。タンパク質クラウンと呼ばれる吸着タンパク質は、NPの表面特性に影響を与える可能性があります。例えば、それらは、NPの表面電荷、凝集特性、および/または流体力学的直径を変える可能性があります。さらに、NP表面へのタンパク質の吸着は、それらのコンフォメーション変化を引き起こし、吸着されたタンパク質の機能的活性を低下または完全に阻害する可能性があります。タンパク質クラウンは、主に、アルブミン、フィブリノーゲン、免疫グロブリンGなどの主要な血清タンパク質、およびその他のエフェクター、シグナル、機能分子で構成されています[88、89]。 NPに結合するとタンパク質構造が変化し、酵素活性の喪失、生物学的プロセスの妨害、およびアミロイド線維などの秩序だった高分子構造の沈殿につながります[90]。これは、アミロイドーシスなどのさまざまな病気につながる可能性があります。インビトロ実験は、親水性ポリマーでコーティングされたQDがヒトβ 2 のフィブリルの形成を加速することを示しました。 ミクログロブリンは、粒子表面で多層構造に配置されます。これにより、NP表面のタンパク質濃度が局所的に増加し、沈殿し、オリゴマーが形成されます[91]。
Xu etal。表面のさまざまな変更を介してNP電荷を負から正に変更する方法を開発しました。たとえば、ポリマーNPはpH感受性ポリマーで修飾されているため、中性媒体では負に帯電し、pH 5〜6の酸性媒体では正電荷を獲得します[92]。この技術は、腫瘍細胞への薬物送達に使用できる、細胞によるNP取り込み速度を大幅に高めることを可能にします。 H9C2、HEK293、A549、およびMCF-7細胞に対する表面修飾酸化セリウムNPの細胞毒性の推定は、NPを正または負に帯電または中性にするために異なるポリマーを使用することにより、基本的に異なる生物学的および毒性効果が得られることを示しています。具体的には、正に帯電したNPと中性のNPはすべての細胞タイプに同じ速度で吸収されますが、負に帯電したNPは主に腫瘍細胞に蓄積します[93]。したがって、NP電荷の変更により、それらの局在化と毒性を制御することができ、化学療法薬を腫瘍に送達するための効果的なシステムを開発するために使用できます。
ナノ粒子の殻と毒性
NPの表面へのシェルの適用は、NPの光学的、磁気的、および電気的特性を変更するために必要です。凝集能力を低下させ、安定性を高めるなどして、NPの生体適合性と水および体液への溶解性を向上させるために使用されます。したがって、シェルはNPの毒性を低下させ、さまざまな種類の細胞との選択的相互作用の能力を提供します。生体分子。さらに、シェルはNPの薬物動態に大きな影響を与え、体内でのNPの分布と蓄積のパターンを変化させます[94]。
上記のように、NPの毒性は主にフリーラジカルの形成に関連しています[40、57、95、96]。ただし、シェルはこの悪影響を大幅に軽減または排除し、NPを安定させ、環境要因に対する耐性を高め、毒性物質の放出を減らし、組織特異的にすることができます[97]。たとえば、Cho etal。それらをレクチンでコーティングすることによって修飾されたポリマーNP。修飾されたNPは、腫瘍細胞と選択的に結合し、表面にシアル酸分子を提示します。これにより、NPは癌細胞を特異的に標識するのに適したものになりました[98]。
NP表面は、有機化合物と無機化合物の両方で修飾できます。たとえば、ポリエチレングリコール、ポリ乳酸、ポリ乳酸、脂質、タンパク質、低分子量化合物、シリコンなどです。このさまざまな修飾因子により、NPの特性を変更したり、特定の輸送や蓄積を行ったりするために、NP表面に複雑なシステムを形成することができます。
合成ポリマーのシェルでコーティングされたナノ粒子は、抗原の送達に使用されるため、免疫応答を高めるアジュバントとして機能します。これにより、強力な自然の非特異的細胞性免疫の標的である抗原に対するワクチンを入手することができます[99]。
金属コアは疎水性であり、主にカドミウム、テルル、水銀などの有毒な重金属で構成されているため、シェルは可溶化を改善し、QDの毒性を低減するためによく使用されます。シェルはQDコアの安定性を高め、その脱塩と酸化または光分解を防ぎます。これにより、QDコアの外側への金属イオンの漏れが減少し、QDの毒性が減少します[100,101,102]。
ナノ粒子毒性の研究
過去20年間で、NPの使用は大幅に拡大し、生物学的および生態学的システムに対するNPの潜在的な毒性効果を研究する新しい科学であるナノ毒性学の基礎につながりました。ナノ毒性学の一般的な目標は、安全なNPの合成規則を開発することです[103]。これには、NPの毒性特性と、細胞、組織、臓器、および体全体に対するそれらの影響を分析するための包括的で体系的なアプローチが必要です。
生体系に対するさまざまな物質の影響を研究するための2つの日常的なアプローチがあり、これらはNP毒性影響にも適用できます。モデル細胞株でのinvitro実験と実験動物でのinvivo実験です。 NPの毒性効果の経路とメカニズムは、コンピュータモデルがNPと生物との間の相互作用の結果を予測するのに十分に知られていないため、ここではNP毒性を推定するための3番目の可能なアプローチであるコンピュータシミュレーションを考慮しません。十分な信頼性を備えた幅広いNP。
NP毒性を研究するための細胞培養モデルと動物実験モデルの両方に、特定の長所と短所があります。前者は、毒性の分子メカニズムへのより深い洞察とNPの主要な標的の同定を可能にします。 however, the patterns of the distribution of NPs in the body and their transport to different tissues and cells are not taken into consideration. The study of NP toxicity in animal experiments allows the delayed effects of NP action in vivo to be estimated. However, the general pattern of toxicity manifestations becomes so complicated that it is impossible to determine which of them is the primary cause of the observed effect and which are its consequences.
Study of Toxicity in Cell Cultures
Many studies of NP toxicity are carried out in primary cell cultures serving as models of various types of human and animal tissues. In some cases, tumor cell lines are used, e.g., for estimating the toxic effects of NPs used in cancer chemotherapy. The type of cells is selected according to the potential route by which NPs enter the body. This may be oral uptake (mainly by ingestion), transdermal uptake (through the skin surface), inhalation uptake of NPs contained in the breathing air, or intentional NP injection in clinic. Intestinal epithelium cells (Caco-2, HT29, and SW480) are often used in experimental models for studying the toxicity of ingested NPs (Table 1). In these models, the kinetics of NP uptake by cells and the viability of cells upon the NP uptake are studied.
<図> 図>The NPs that serve as carriers of drugs or contrast agents, or those used for imaging, are administered by injection. The toxicity of these NPs is studied in primary blood cell cultures. Most commonly, hemolysis, platelet activation, and platelet aggregation are estimated. In addition to primary blood cell cultures, cultured HUVECs, mesenchymal stem cells, mononuclear blood cells, and various tumor cell lines (HeLa, MCF-7, PC3, C4-2, and SKBR-3) are used (Table 2).
<図> 図>The toxicity of inhaled NPs is studied using the cell lines modeling different tissues of the respiratory system, e.g., A549 and C10 cells of pulmonary origin, alveolar macrophages (RAW 264.7), various epithelial cells and fibroblasts (BEAS-2B, NHBE, 16-HBE, SAEC), as well as human monocytes (THP-1) (Table 3).
<図> 図>The toxicity of NPs that enter the body transdermally is usually studied in keratinocytes, fibroblasts, and, more rarely, sebocytes (cells of sebaceous glands) (Table 4).
<図> 図>Co-cultured Cell Lines and 3D Cell Cultures
Although the majority of in vitro nanotoxicity studies are carried out on cell monocultures, studies using two other approaches are increasingly often reported in the literature. One of them is co-culturing of several types of cells; the other is the use of 3D cultures. The rationale for these approaches is the need for more realistic models of mammalian tissues and organs. For example, co-cultured Caco-2 epithelial colorectal adenocarcinoma cells and Raji cells (a lymphoblast cell line) have served as a model of the human intestinal epithelium in experiments on the toxicity of silver NPs [104]. A co-culture of three cell lines derived from lung epithelial cells, human blood macrophages, and dendritic cells has been used as an experimental model in a study on the toxic effects of inhaled NPs [105]. A model of skin consisting of co-cultured fibroblasts and keratinocytes has been suggested [106].
It is known that the cell phenotype, as well as cell functions and metabolic processes, is largely determined by the complex system of cell interactions with other cells and the surrounding extracellular matrix [107]. Therefore, many important characteristics of cells with an adhesive type of growth in a monolayer culture substantially differ from those of the same cells in the living tissue; hence, conclusions from many experiments on the NP toxic effects on cells growing in a monolayer are somewhat incorrect [108]. Experimental 3D models of tissues and organs have been used for analysis of NP toxicity and penetration into cells in several published studies. For example, there are 3D models based on polymer hydrogels [109] and models constructed in special perfusion chambers containing a semipermeable membrane to which the cells are attached. Li etal。 and Lee et al. [110, 111] used multicellular spheroids about 100 μm in size to obtain a 3D model of the liver and compare the toxicities of CdTe and Au NPs in experiments on this model and a monolayer culture of liver cells [111]. The results obtained using the 3D model were more closely correlated with the data obtained in experiments on animals, which indicates a considerable potential of this approach for adequate and informative testing of NP toxicity.
In vivo Study of Nanoparticle Toxicity
In addition to the study of multilayered and 3D cell cultures, the behavior of NPs in the living body is being extensively studied. Since these studies are focused on the biomedical applications of NPs, the NP toxicity for living organisms remains an important issue. Although NPs are highly promising for various clinical applications, they are potentially hazardous. This hazard cannot be estimated correctly in vitro, following from the comparison of the in vivo and in vitro effects of NPs.
Titanium dioxide (TiO2 ) particles are among the most widely used NPs, in particular, in environment protection measures. Therefore, it was exceptionally important to estimate their toxicity in the case of a 100% bioavailability, namely, in experiments with their intravenous injection to experimental animals. This study has been performed by Fabian et al. [112]. Experimental animals (rats) were injected with a suspension of TiO2 NPs at a dose of 5 mg/kg, and their biodistribution, as well as the general condition of the animals, was monitored. The results have shown that the animals exhibit no signs of ailment or disorder, nor is inflammation or another manifestation of a toxic effect observed, within 28 days. This suggests that TiO2 NPs are relatively harmless.
Silver NPs are another example of NPs potentially useful in medicine, owing to their antimicrobial activity. Their toxicity and biodistribution were analyzed in an experiment where CD-1 mice were intravenously injected with 10 mg/kg of silver NPs of different sizes (10, 40, and 100 nm) coated with different shells. Although each type of NPs was found to cause toxic damage of tissues, larger particles were less toxic, probably, due to their lower penetration capacity [113]. Asare et al. [114] estimated the genotoxicity of silver and titanium NPs administered at a dose of 5 mg/kg. They have found that silver NPs cause DNA strand breaks and oxidation of purine bases in the tissues examined. Gold nanoparticles have a similar effect [115]. They have been shown to be toxic for mice, causing weight loss, decrease in the hematocrit, and reduction of the red blood cell count.
Targeted drug delivery is one of the most important applications of NPs. In this case, it is also paramount to know their toxic properties, because the positive effect of their use should prevail over the negative one. Kwon et al. [116] have developed antioxidant NPs from the polymeric prodrug of vanillin. Their study has shown that the NPs have no toxic effect on the body, specifically the liver, at doses lower than 2.5 mg/kg. Similar results have been obtained for gelatin NPs modified with polyethylene glycol, which are planned to be used for targeted delivery of ibuprofen sodium salt [117]. The NPs have proved to be nontoxic at the dose that is necessary for effective drug delivery (1 mg/kg), which has been confirmed by measuring the inflammatory cytokine levels in the animals studied, as well as histological analysis of their organs.
Quantum dots are among the NPs that are most promising for medical applications (Fig. 2). However, they are potentially hazardous for human health, because they exhibit various toxic effects in both in vitro and in vivo experiments [118,119,120,121,122].
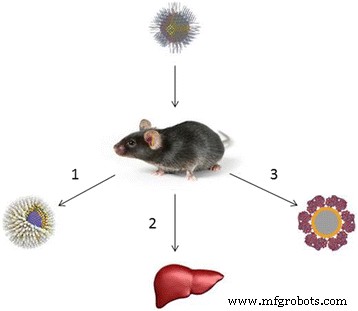
The possible reasons why quantum dots may be nontoxic in animal models. (1) The shell prevents the leakage of heavy metals into the body [129, 135]. (2) Quantum dots are localized in the liver and subsequently eliminated from the body [135, 173]. (3) The protein crown around quantum dots protects the body from heavy metals [132, 174]
Toxic effects of QDs in vivo are usually studied in experiments on mice and rats [123]. A study on the toxicity of cadmium-based QDs for mice showed that QDs were distributed throughout the body as soon as 15 min after injection to the caudal vein, after which they accumulated in the liver, kidneys, spleen, red bone marrow, and lymph nodes. Two years after the injection, fluorescence was mainly retained in lymph nodes; in other organs, no QDs were detected [124]. It should be also noted that the fluorescence spectrum was shifted to the blue spectral region because of the destruction of the QD shell and changes in the shape, size, and surface charge of the QDs. This, however, occurred rather slowly, because the QDs were found to be nontoxic after their injection at the doses at which pure cadmium ions would have had a lethal effect. Similar results were obtained by Yang et al. [125].張ら。 [95] showed that CdTe QDs predominantly accumulated in the liver, decreasing the amount of antioxidants in it and inducing oxidative stress in liver cells.
Cadmium and tellurium ions tend to accumulate in various organs and tissues upon degradation and decay of the cores of CdTe/ZnS QDs. Experiments on mice have shown that cadmium predominantly accumulates in the liver, kidneys, and spleen, whereas tellurium accumulates almost exclusively in the kidneys [126]. Ballou et al. [127] found that cadmium-containing QDs coated with polymer shells of polyacrylic acid or different derivatives of polyethylene glycol had no lethal effect on experimental mice and remained fluorescent for 4 months. СdSe/ZnS NPs also had no detectable pathological effect on mice [128]; however, the absence of distinct signs of pathology still does not mean that the QDs are absolutely nontoxic.
Hu etal。 [129] found that lead-containing QDs had no toxic effect on mice for 4 weeks; however, this was most probably because the QDs studied were coated with a polyethylene glycol shell.
Since heavy metals contained in QDs are a factor of their toxicity, several research groups suggested that heavy-metal-free NPs be synthesized. For example, Pons et al. [130] synthesized CuInS2/ZnS QDs fluorescing in the near-infrared spectral region (at a wavelength of about 800 nm) and supposed that this composition would make the QDs nontoxic for experimental animals. Comparison of the effects of CuInS2 /ZnS and CdTeSe/CdZnS QDs on regional lymph nodes in mice showed that the lymph nodes were only slightly, if at all, enlarged upon injection of the QDs not containing heavy metals, whereas injection of the CdTeSe/CdZnS QDs induced a distinct immune response in them [130]. QDs in which silicon was substituted for heavy metals also had no toxic effect on mice [131].
Even QDs containing heavy metals are often found to be nontoxic. One of the possible explanations is that QDs are coated with the protein crown upon entering the living body; this crown shields their surface and protects cells against damage [132]. Usually, the proteins that are included in the NP molecular corona are major serum proteins, such as albumin, immunoglobulin G (IgG), fibrinogen, and apolipoproteins [133]. Molecular corona also can influence on the interaction of NPs with cells. Zyuzin et al. have demonstrated that, in human endothelial cells, the NP protein corona decreases the NP nonspecific binding to the cell membrane, increases the residence time of NP in early endosomes, and reduces the amount of internalized NPs [134].
However, even in the absence of direct signs of intoxication in experimental animals, it remains unclear whether the use of QDs in medicine is safe for humans. In some cases, the QD toxicity was not detected in mice because the NPs were neutralized by the liver and accumulated in it [135]; in other cases, QDs coated with phospholipid micelles exhibited reduced toxicity owing to the shell [129]. Despite the extensive in vivo studies on QD toxicity, their use in biomedicine remains an open question. One of the main reasons is that all the delayed effects of QDs cannot be monitored in experimental animals, because their lifespan is as short as a few years, which is insufficient for complete elimination or degradation of NPs.
結論
The potential toxicity of NPs is the main problem of their use in medicine. Therefore, not only positive results of the use of NPs, but also the possible unpredictable negative consequences of their action on the human body, should be scrutinized. The toxicity of NPs is related to their distribution in the bloodstream and lymph stream and their capacities for penetrating into almost all cells, tissues, and organs and interacting with various macromolecules and altering their structure, thereby interfering with intracellular processes and the functioning of whole organs. The NP toxicity strongly depends on their physical and chemical properties, such as the shape, size, electric charge, and chemical compositions of the core and shell. Many types of NPs are not recognized by the protective systems of cells and the body, which decreases the rate of their degradation and may lead to considerable accumulation of NPs in organs and tissues, even to highly toxic and lethal concentrations. However, a number of approaches to designing NPs with a decreased toxicity compared to the traditional NPs are already available. Advanced methods for studying the NP toxicity make it possible to analyze different pathways and mechanisms of toxicity at the molecular level, as well as reliably predict the possible negative effect at the body level.
Thus, it is obvious that designing NPs that have small or no negative effects is impossible unless all qualitative and quantitative physical and chemical properties of NPs are systematically taken into consideration and a relevant experimental model for estimating their influence on biological systems is available.
略語
- FDA:
-
食品医薬品局
- IL-1β:
-
Interleukin-1-beta
- MRT:
-
Magnetic resonance tomography
- NP:
-
Nanoparticle
- QD:
-
量子ドット
- ROS:
-
活性酸素種
- SEM:
-
走査型電子顕微鏡
- TEM:
-
透過型電子顕微鏡
- TNFα:
-
Tumor necrosis factor alpha
ナノマテリアル