強化された薬物送達のための喉頭癌細胞および免疫細胞内の高分子ナノ粒子の細胞内在化の定量的評価
要約
ポリ(乳酸-co-グリコール酸)(PLGA)ベースのナノメディシンの臨床翻訳は、食細胞による非特異的食作用に起因する不十分な送達効率のために、制限されています。癌細胞と免疫細胞の間のナノ粒子の相互作用を理解することは、ほとんどとらえどころのないままです。この研究では、単球/マクロファージの有無にかかわらず、単一培養または共培養システムで喉頭癌細胞に対する蛍光PLGA粒子(100 nm、500 nm、および1 µm)の細胞内在化に関する定量的調査を最初に実施しました。 5〜20 µg / mLの濃度のPLGA粒子は、500nmおよび20µg / mLの1µm PLGA粒子が細胞生存率をわずかに低下させることを除いて、優れた生体適合性を示します。顕微鏡観察により、3つのサイズの粒子すべてが癌細胞とマクロファージの両方によって効果的に摂取されていることがわかりました。しかし、定量的蛍光検査により、癌細胞の取り込み指数(マクロファージあたりの癌細胞あたりの平均細胞内粒子蛍光に正規化された細胞あたりの平均細胞内粒子蛍光)は、単一培養の場合と比較して、共培養のすべてのPLGA粒子で大幅に低下することが明らかになりました(1.35〜1.05、 100 nm、500 nm、および1 µmの粒子の場合はそれぞれ1.50〜0.59、および1.4〜0.47)。フローサイトメトリーを使用した定量分析により、共培養における癌細胞の取り込み指数の低下がさらに確認されましたが、マクロファージあたりの粒子数は多くなりました。マクロファージの融合を介した多核巨細胞の形成は、PLGA治療後に増加することもわかっており、これは腫瘍薬物送達の潜在的なアプローチとしてさらに活用できる可能性があります。全体として、これらの発見は、ナノ粒子免疫癌細胞の相互作用への新しい洞察を提供し、喉頭癌の治療のためのPLGAベースのナノキャリアの適用を容易にする可能性があります。
はじめに
癌は世界の主要な死亡原因の1つであり、2018年には約1,000万人の新たな死亡例が報告されています[1]。喉頭がん(喉頭から発生するがん細胞)は、頭頸部扁平上皮がん(HNSCC)の2番目に多い悪性腫瘍であり、2018年には約18万人の新規症例と95,000人の死亡を占めています[2]。現在、標的薬は、外科手術、放射線療法、化学療法などの従来の治療法によって導入された課題に対するオプションの治療法として開発されています[3]。たとえば、新規の薬物ナノキャリア(すなわち)を開発することにより、薬物の標的化と有効性を改善し、望ましくない副作用を減らすための科学的取り組みが促進されています。 、マイクロニードル)、個別化された抗がん剤、および治療用抗体を標的としたデリバリーシステム[4]。
ナノキャリアは、シスプラチン、パクリタキセル、ドセタキセルなどの抗がん剤をロードするために広く利用されており、水溶性、バイオアベイラビリティ、安定性を向上させて、薬物送達と有効性を向上させています[3、4]。一般に、ナノ粒子(NP)ベースのドラッグデリバリーは、他のさまざまな物質のデリバリーを可能にします(例 、タンパク質、抗体、ワクチン、および核酸)は、動物モデルと患者の両方の体の特定の領域に適用されます[4、5]。しかし、いわゆる強化された透過性と保持(EPR)効果により、さまざまな種類の癌間でターゲティング効率が大幅に異なります[6]。最近の証拠は、腫瘍へのナノドラッグキャリア(ここでは金のNP)の沈着は、トランスサイトーシスのプロセスに優先的に依存することを示唆しています[7]。もう1つは、エンドサイトーシス、小胞移動、およびエキソサイトーシスのコースを含みます。ただし、標的化薬物送達のメカニズムに関する矛盾した結果は、invitro細胞培養からexvivo組織培養に至るまでの複数の実験戦略を利用したナノ薬物またはNPとの細胞相互作用の基礎を理解するための非常に重要なことを強調していることに注意する必要がありますおよびinvivo動物実験。
リポソーム、アルブミンNP(NP)、シリカNP、およびポリ(乳酸-co-グリコール酸)(PLGA)のような多数のナノキャリアが、喉頭癌を含むさまざまな種類の癌を治療するために臨床的に使用されてきました[4]。ミセルやPLGAなどのポリマーベースのNPは、単純な混合または共有結合によるナノ薬物の多様な製剤、自己組織化の優れた能力、薬物負荷の高い能力、および生体適合性により、生物医学的用途で大きな可能性を秘めています[8、9 ]。たとえば、ドキソルビシンとインドシアニングリーンをロードしたポリエチレングリコール(PEG)でコーティングされたPLGAナノキャリアは、乳がんの相乗的な化学光熱療法を可能にします[10]。また、invitroおよびinvivoの両方の研究で、シスプラチンをロードしたミセルは、HNSCC同所性腫瘍に対して優れた抗癌活性を示すことが示されています(すなわち 、SAS-L1およびHSC-2)[11]。 PLGAやミセルなどのいくつかのポリマーベースのナノドラッグキャリアは、臨床使用が承認されているか、臨床試験で評価されています[4、6]が、さらに多くのポリマーナノドラッグが、さまざまな頭頸部がん細胞で前臨床試験中です。動物の系統および異種移植腫瘍モデル[11、12、13、14]。
科学的証拠は、ナノ薬物担体に対する宿主の免疫応答の重要性を浮き彫りにしました。なぜなら、これらのNPが一度体内に入ると、免疫系によって不可避的に認識されるようになるからです。マクロファージは、アレルゲン、微生物、および異物の中和と除去に特化した、細胞宿主防御の第一線と見なされています(例 、ナノキャリア)食作用およびその結果としての免疫応答のプライミングを介して。新規に設計されたナノキャリアのほとんどは、肝臓のクッパー細胞や脾臓の赤脾髄マクロファージなどの単核食細胞系(MPS)にNPが効率的に蓄積するため、invivoで特定の疾患領域または腫瘍への送達を標的にすることができませんでした。 [15]。したがって、単球やマクロファージなどの免疫細胞によるNPの細胞取り込みのメカニズムを理解することは、関連する組織や体液中のナノキャリアの寿命を決定するため、非常に重要です。したがって、新しいin vitro細胞共培養システムは、in vivo条件をより適切に反映できる、より意味のある結果を達成するための需要が高まっているため、ナノメディシンおよび毒物学の分野で徐々に注目を集めています[16]。実際、共培養システムは、健康な組織状態や病気の組織状態を模倣する現実的な状況を示すことが証明されており[17]、NP細胞の取り込みと薬物吸収の研究で確実に利用されています[18、19、20、21]。癌細胞と免疫細胞の共培養モデルの利用は、一般に、これらのナノ材料の細胞への取り込み経路とメカニズムを精査するための適切なプラットフォームを提供します。したがって、免疫細胞の存在下での癌細胞におけるNPの取り込み効率と運命を決定することは非常に重要です。ほとんどのナノキャリアは、EPR効果を利用して血液循環を延長するために、直径50〜200 nmになるように設計されていますが、より大きなNP(> 500 nm)はMPSによって効率的に除去されると報告されています[6]。したがって、さまざまなサイズ(100、500、および1000 nm)のFDA承認ナノキャリアであるPLGAを選択して、UM-SCC-17A(古典的な喉頭扁平上皮癌細胞株)[22]およびTHP-の取り込み能力を調査しました。 1(ヒト急性単球細胞株)細胞。いくつかのinvitro研究では、PLGAナノキャリアを適用して単培養でHNSCC癌細胞を殺す薬剤を送達しました[12、13、23]。これは、免疫細胞と喉頭癌細胞によるNP取り込みの取り込み効率とメカニズムを同期的に調べる最初の研究です。さまざまなサイズのPLGAを採用した共培養モデル。これは、HNSCC療法用の新しい安全な設計によるナノメディシンの開発の基本的な基盤を提供する可能性があります。
材料と方法
資料
この研究では、100 nm、500 nm、1000 nmを含むさまざまなサイズの3つの市販のPLGA粒子(Sigma-Aldrich)を使用しました。すべての粒子には、460nmと500nmの光励起および発光(ex / em)波長を持つ緑色のフルオロフォアがロードされました。すべての粒子は粉末の形で受け取られ、さらに使用するために最終濃度が10 mg / mLの蒸留水に懸濁されました。 3つの粒子の流体力学的直径とゼータ電位は、Malvern Zeta Sizer Nano装置(Malvern Instruments Ltd.、Malvern、UK)を使用して実施された動的光散乱(DLS)によって実行されました。各粒子のストック懸濁液は、DLS測定用に80 µlの蒸留水で1:100に希釈されました。メーカーからの指示に従い、各粒子について3回の繰り返し測定が行われました。
UM-SCC-17AおよびTHP-1の細胞培養と粒子曝露
米国のSigma-AldrichおよびShanghaiHengya Biotechnology Company(中国の上海)からそれぞれ購入したヒト喉頭癌細胞株UM-SCC-17Aおよびヒト単球/マクロファージ細胞株THP-1を使用して、invitroモデルを構築しました。この研究。 UM-SCC-17A細胞およびTHP-1細胞は、DMEM [22]または10%FBS(Gibco、ドイツ)および1%ペニシリン-ストレプトマイシン溶液(Gibco、ドイツ)を添加したRPMI-1640細胞培養培地で37℃で培養しました。 5%CO2で°C。 THP-1細胞を100nM Phorbol 12-myristate 13-acetate(PMA)(Sigma、USA)溶液に72時間曝露した後、細胞を播種してマクロファージに分化させました。両方の細胞は、3日ごとに0.5%トリプシン-EDTAを使用して継代され、細胞の健康状態を確認するために細胞の形態が毎日チェックされました。
細胞を24ウェルプレートに0.1×10 6 の密度で播種しました WST-1およびLDHアッセイ用の単培養細胞の場合は細胞/ウェル、蛍光顕微鏡用の24ウェルプレートの滅菌ガラスカバースリップ上。共培養モデルでは、UM-SCC-17A細胞を最初に24ウェルプレートに50,000 /ウェルの密度で一晩播種し、次に50,000 /ウェルTHP-1細胞を添加しました。粒子は、単培養UM-SCC-17AおよびTHP-1細胞の場合はそれぞれの細胞培養培地または共培養細胞の場合は1:1混合細胞培養培地のいずれかで、500 µlに懸濁し、細胞サンプルに24時間曝露しました。 WST-1およびLDHアッセイの場合は5、10、および20 µg / mL、蛍光顕微鏡の場合は10 µg / mLの濃度。
FACS測定では、前述と同じ方法で、細胞を12ウェルプレートに250,000細胞/ウェル、または共培養モデルの場合はそれぞれ125,000細胞/ウェルの密度で播種しました。細胞を1mLの細胞培養培地で一晩増殖させ、最終濃度10 µg / mLのPLGA粒子に24時間曝露しました。
細胞生存率アッセイ
細胞生存率は、細胞増殖試薬WST-1キット(Roche、ドイツ)によって、製造元の指示に従って決定されました。簡単に説明すると、WST-1溶液を、単一培養UM-SCC-17AまたはTHP-1の場合はそれぞれの細胞培養培地で、または共培養細胞の場合は1:1混合細胞培養培地で1:10に希釈しました。曝露後、上清を排出し、細胞をWST-1アッセイの500 µlの作業溶液とともに37°Cで30分間インキュベートしました。サンプルを収集し、14,000 rpmで10分間遠心分離して、粒子を除去しました。溶液の吸光度(OD値)は、Infinite ® を使用して450nmの波長で測定されました。 F200(テカン、米国)。 WST-1作業溶液のみを含むブランクサンプルの値を差し引くことにより吸光度値を補正し、相対的な細胞生存率を未処理のコントロールサンプルと比較しました。
細胞膜漏出アッセイ
乳酸デヒドロゲナーゼ(LDH)の放出は、市販の細胞毒性検出キット(LDH)(Roche、Germany)を使用して測定し、PLGA粒子による細胞毒性を測定しました。細胞の上清を曝露の24時間後に収集し、14,000 rpmで10分間遠心分離し、200 µlの完全細胞培養培地で1:10に希釈しました。ポジティブコントロールは、細胞を0.2%Triton X-100とともに37°Cで15分間インキュベートし、200 µlの完全細胞培養培地で1:50に希釈することによるLDHの総放出量として定義されました。サンプルをLDHアッセイの100の作業溶液と室温で30分間インキュベートし、50 µlの1%HClを使用して反応を停止しました。 Infinite®F200(Tecan、USA)を使用して、波長492 nmでの吸光度を測定し、次の式に従って相対LDH濃度を計算しました
$$ {\ text {Relative}} \; {\ text {LDH}} \; {\ text {concentration}} =\ left({{\ text {sample}} \; {\ text {OD}} {- } {\ text {blank}} \; {\ text {OD}}} \ right)/ \ left({{\ text {positive}} \; {\ text {control}} \; {\ text {OD} } {-} {\ text {blank}} \; {\ text {OD}}} \ right)\ times 5 \ times 100 \%。$$蛍光顕微鏡
細胞は、蛍光顕微鏡下での粒子の局在化のためにヘキスト染色によって視覚化された。粒子を24時間曝露した後、細胞をPBSで3回洗浄し、4%ホルムアルデヒドとともに室温で10分間インキュベートしました。ホルムアルデヒドで固定した後、細胞を1:1000に希釈した Hoechst を含む200µlの染色液でインキュベートしました。 および1%BSAを含むPBSで30分間。次に、カバーガラスを逆さまにスライドガラス上に移動し、視覚化のためにDAKO蛍光退色防止剤の液滴で維持しました。細胞形態用の明るいフィールド、細胞核用のDAPI、および粒子用のGFPを含む、4つの光チャネルを蛍光顕微鏡で設定しました。各蛍光画像の粒子チャネルの露光時間を記録し、さまざまな粒子にわたる蛍光強度の均質化に使用し、ImageJ(https://imagej.nih.gov/ij)によってランダムに選択された領域を使用して蛍光強度によって細胞内粒子を計算しました。 /)。異なる粒子間の取り込み指数を、単培養または共培養のUM-SCC-17A細胞間で比較しました。簡単に言えば、内在化された粒子の平均蛍光強度(MFI)は、たとえば、各細胞タイプについて50細胞で計算され、これは、総蛍光強度( I 合計 )特定の領域と自家蛍光( I auto )粒子のない領域の方程式[24、25]の同じサイズの領域。癌細胞の取り込み指数は、単培養モデルまたは共培養モデルのいずれかでTHP-1細胞のMFIに正規化されたUM-SCC-17AのMFIによって決定されました。計算は次の式に従って実行されました:
$$ {\ text {uptake}} \; {\ text {index}} =\ frac {{I _ {{{\ text {total1}}}} --I _ {{{\ text {auto1}}}} \ left({{\ text {MFI}} _ { {{\ text {UM}}-{\ text {SCC}}-17A}}} \ right)}} {{I _ {{{\ text {total2}}}}-I _ {{{\ text {auto2} }}} \ left({{\ text {MFI}} _ {{{\ text {THP}}-1}}} \ right)}} $$蛍光活性化セルソーティング(FACS)
蛍光活性化セルソーティング(FACS)を実行して、3つの粒子の取り込み能力を測定しました。細胞をPBSで3回洗浄して、曝露の24時間後に細胞培地と解離性粒子を除去し、200 µlの0.5%トリプシン-EDTA(Gibco、ドイツ)と37°Cで4分間インキュベートして、プレートから細胞を除去しました。 。次に、2 mlの完全な細胞培養培地を加えて反応を停止し、細胞懸濁液をFACS測定用のガラス管に移し、300×G、4°Cで5分間遠心分離しました。上清を穏やかに排出し、細胞を200 µlのPBSに懸濁し、氷上で保存しました。生細胞は、最初に、前方(FSC-A)側(SSC-A)散乱を使用して、破片と死細胞からゲート制御されました。次に、共培養細胞をAPCチャネルとFSC-Aを使用して分析し、細胞のサイズに基づいてマクロファージからUM-SCC-17A細胞を分離しました。各サンプルについて合計30,000個の細胞を分析し、各細胞の平均蛍光強度を計算して、異なる粒子間で正規化しました。一方、細胞と粒子を24時間インキュベートした後、細胞培養上清、洗浄バッファー(細胞表面に付着した残留粒子を除去するために、3回洗浄)、および細胞(トリプシン処理消化)を収集し、マイクロプレートを使用して蛍光強度を測定しました。リーダー(Infinite®F200、Tecan)。このアプローチにより、細胞が摂取する粒子の割合をグループごとに決定できます。たとえば、100nmの5µgの線量で12ウェル/プレート(合計250,000細胞)の単一細胞に曝露された約30,000個の粒子PLGA粒子は、単一の細胞によって内在化された平均13,000の粒子をもたらします。これは、細胞に送達された適用用量の約43%です。この配信されたパーセンテージをさらに使用して、FACSの各タイプの細胞の共培養システムでの粒子数を計算できます。
細胞融合の定量化
多核巨細胞(MGC)へのマクロファージ融合は、正常な細胞質内に2つ以上の核を形態学的に含む巨細胞として定義され、文献に記録されているように、染色後の明視野画像および蛍光画像で明確かつ同期的に識別できます[26]。 。細胞融合のパーセンテージは、ImageJ(https://imagej.nih.gov/ij/)の自動化されたアプローチで決定された、総細胞数に正規化されたMGC核(手動で数える)の数によって計算されました。
統計分析
GraphPad V8.0ソフトウェア(GraphPad Software Inc.、米国カリフォルニア州サンディエゴ)を統計分析と結果の視覚化に使用しました。一方向ANONAに続いて、Holm-Sidak法またはt検定のいずれかを実行して、複数グループの結果または2グループの結果をそれぞれ比較しました。すべての実験は独立した三重化で実施され、データは平均±標準偏差(STD)として表されました。 p の結果 値<0.05(*)および p <0.01(**)は有意であると見なされました。
結果と考察
ポリ(乳酸-co-グリコール酸)粒子の特性評価
PLGA粒子の蛍光顕微鏡写真(図1a、b、c)は、強い蛍光強度を示し、調製後14日間にわたって蛍光シグナルの有意な減少は見られませんでした(追加ファイル1:S1)。これは、比較的均一なサイズ分布と高い蛍光を示唆しています。安定。サプライヤーが示したように、100、500、および1000 nmのPLGA粒子は、それぞれ約80.6±19.3 nm、542.6±128.3 nm、および951.9±237.5 nmのサイズを示しました(図1d、e、f)。ゼータ電位測定によると、粒子の平均表面電荷は、100、500、および1000の多分散度指数が0.057、0.056、および0.062で、-20.6±5.3、-17±4.6、および-16.5±3.5であることがわかりました。それぞれnm粒子であり、後者は高度に単分散の標準を示しています。すべての粒子をボルテックスした後、ウォーターバスで5分間超音波処理して、粒子の凝集を大幅に排除しました。ただし、非常に少量(約3〜4%)の粒子の凝集が避けられないため、直径が約4〜6 µmの100および1000nmの粒子に対応する小さなピークが観察されました。
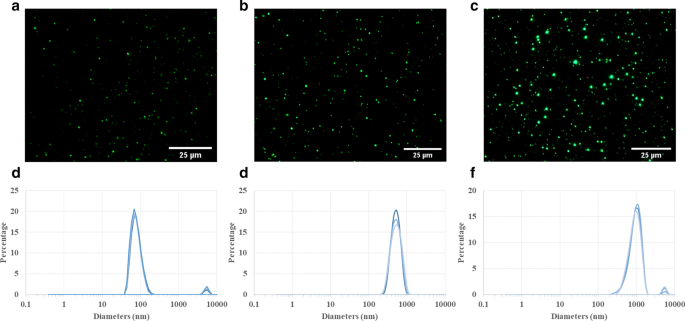
PLGA粒子の特性評価。蛍光顕微鏡写真はさまざまなサイズを示しています( a 100 nm、 b 500 nm、および c 1 µm)のPLGA粒子。これらの粒子は、オリンパス光学顕微鏡で検出でき、水懸濁液中で比較的均一な粒子サイズ分布を示します。動的光散乱(DLS)測定では、100 nm( d )の体積で重み付けされたサイズ分布が表示されます )、500( e )、1 µm( f )PLGA粒子。 100nmおよび1µmのPLGA懸濁液では、粒子凝集のごく一部(小さいピーク:3〜4%)にもかかわらず、メインピークが狭いため、全体的な粒子サイズ分布は非常に均一です。
細胞の生存率と細胞毒性の評価
PLGA粒子は、その優れた生体適合性と生分解性により、FDAおよび欧州医薬品庁によって生物医学的用途で承認されています[27、28]。したがって、PLGA粒子は現在、診療所で使用されており、特定の疾患領域または腫瘍に薬物を送達するナノキャリアとして前臨床試験に広く適用されています。ただし、PLGA粒子のターゲティング効率と治療効果は、少なくとも部分的には免疫系によって妨げられます。たとえば、肝臓のクッパー細胞による高粒子食作用は、ナノ薬物担体が腫瘍部位に入るのを大幅に制限しました[15]。したがって、癌細胞、免疫細胞、および粒子間の相互作用を研究して、薬物送達のインビボ状況をよりよく模倣するために、高度なインビトロモデルを確立することが極めて重要である。 UM-SCC-17Aは、原発性喉頭がん検体から分離されたユニークな喉頭扁平上皮がん細胞株です[29]。ただし、共培養システムでのin vitro細胞取り込み効率に関する情報(例 、マクロファージと癌細胞の共培養)はまだ不十分であり、invivo応答の予測能力を改善するために対処する必要がある問題です。さらに、粒子サイズおよび表面コーティングは、動物モデルおよび細胞培養における固形腫瘍、患部、および癌細胞への送達能力において重要な役割を果たすことが証明されている[30、31、32、33]。ここでは、このように3つのサイズのPLGAをUM-SCC-17A癌細胞に適用して、単一培養および共培養システムにおける細胞取り込みと細胞内分布に対する粒子サイズの影響を調査しました。
この研究では、単作THP-1にWST-1(4- [3-(4-ヨードフェニル)-2-(4-ニトロフェニル)-2H-5-テトラゾリオ] -1,3-ベンゼンジスルホネート)法を使用して細胞生存率を測定しました。およびUM-SCC-17A細胞、ならびに両方のタイプの細胞の共培養。テストしたすべての濃度で100および500nmのPLGAで処理したグループでは明らかな細胞死は発生しませんでしたが、最高濃度(20 µg / mL)の500nmおよび1µmのPLGA粒子は、単培養UM-SCC-17Aの細胞生存率を大幅に低下させました。細胞(図2a)。予想どおり、THP-1細胞の生存率は、5〜20 µg / mLの濃度で3種類すべてのPLGAと24時間インキュベーションした後でも、大きな影響を受けませんでした(生存率が100%と見なされる未処理の細胞と比較して95%以上の生存率) 、図2b)。単一培養UM-SCC-17A細胞の結果と同じように、共培養システムでの細胞生存率は、ここで使用した3つの投与量で100および500 nmのPLGA粒子が存在しても変化しませんでしたが、20で処理したグループでは大幅に低下しました。 µg / mL 1 µm PLGA(図2c)。
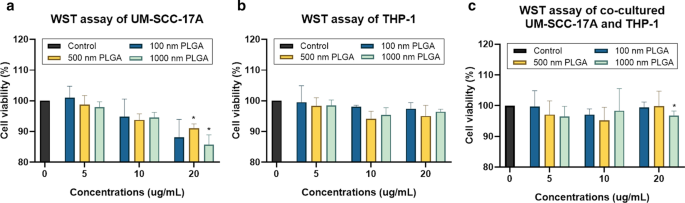
WSTアッセイを利用した単培養および共培養細胞における細胞生存率の決定。 UM-SCC-17A( a )およびTHP-1セル( b )、および共培養されたUM-SCC-17AおよびTHP-1細胞( c )3つのサイズのさまざまな濃度(5〜20 µg / mL)のPLGA粒子で処理しました
LDHアッセイを利用した粒子の細胞毒性の評価は、細胞外LDHの量を測定することによって細胞膜の漏出を測定することです[9]。この細胞質酵素の細胞培養上清への放出は、細胞膜損傷の特徴であり、不可逆的な細胞死をもたらします。 1 µm PLGAのさまざまな用量で処理された単培養UM-SCC-17A細胞では、より高い用量でLDHレベルがわずかに上昇しましたが、20 µg / mLの最高濃度でも細胞に対する明確な細胞毒性は観察されませんでした(図3a)。 。当然のことながら、ここでさまざまな投与量で使用された3つのサイズのPLGAはすべて、上清への実質的なLDH放出を誘導できず、単培養THP-1細胞に対する毒性効果がわずかであることを示しています。これは、上記の細胞生存率の結果とも非常に一致しています(図。3b)。共培養実験では、濃度の異なるすべての粒子が、放出されたLDHレベルに関して、両方の細胞に対して優れた生体適合性を示しました(図3c)。一般に、ここで使用された100nmから1µmの広い粒子サイズ範囲は、リポソーム、ミセル、デンドリマー、ポリマー、ミニセルなどのナノキャリアの一般的なサイズ(50〜200 nm)をカバーします。この範囲には、サブミクロンサイズの粒子も含まれます(500 nmの粒子は、依然としてNPと見なすことができます。例 サイズが約500nmの粒子は、肺内で10〜100nmのNPと同じクリアランス経路を持ち[34]、ミクロンサイズの1 µmです。これらのPLGA粒子はいずれも、UM-SCC-に対して最高濃度の500nmおよび1µm PLGA粒子を除いて、単一培養および共培養システムでTHP-1および/またはUM-SCC-17A細胞に対して明らかな細胞毒性を示しませんでした。 17Aと共培養細胞(ただし、細胞の85%以上が生き残っています)は、これらが薬物送達システムでのアプリケーションに適していることを示しています。
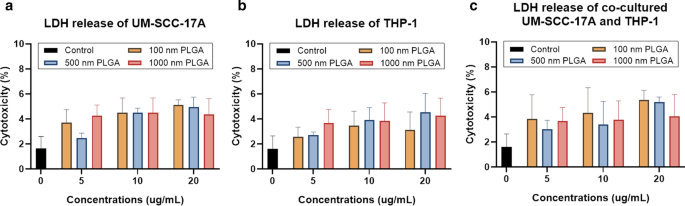
LDHアッセイを使用した単培養および共培養細胞に対する細胞毒性の決定。 UM-SCC-17A( a )およびTHP-1セル( b )、および共培養細胞( c )PLGA粒子で処理されました。粒子(ここではさまざまなサイズと濃度を使用)と細胞を単一培養システムと共培養システムの両方で24時間培養した後、有意な細胞死は観察されませんでした
単一培養および共培養システムにおけるPLGAの細胞取り込み能力
図4は、細胞形態(図4a、d、g)、細胞核、100 nm NP(図4b、e、h)、および単一培養と共培養における拡大画像(図4c、f、i)の統合を示しています。文化システム。対照群の未処理細胞では粒子は観察されませんでした(追加ファイル1:S2)。マクロファージ単培養細胞の細胞質に、巨大な大型の100 nm PLGA NPの凝集体が観察されました(図4c)。一方、単一培養UM-SCC-17Aは、細胞膜内で観察された明るい緑色の蛍光シグナルによって証明された、100 nm PLGAの優れた取り込み能力を示しました(図4f)。 THP-1またはUM-SCC-17A細胞におけるPLGA粒子の細胞内蓄積と、共培養における細胞外粒子をよりよく説明するために、追加ファイル1:S3)のように明視野画像と蛍光画像のオーバーレイを適用しました。共培養システムでは、両方の細胞タイプは、マクロファージが丸い形と小さいサイズを示したのに対し、癌細胞は大きいサイズと細長い形を示した明視野画像の形態と細胞サイズの点で区別できます。ただし、共培養システムでのマクロファージによる100 nm PLGAの高い取り込みにより、癌細胞は不十分な細胞摂取を示しました。これは、マクロファージが、外来微生物、アレルゲン、および粒子を除去するための食作用機能を実行するための効率的で特定のタイプの免疫細胞と見なされているためです。同様に、図5と図6は、それぞれ単細胞培養または混合細胞培養における500nmおよび1µmPLGA粒子の細胞取り込み能力の定性分析を示しています。ここで使用した10mg / mLの濃度のさまざまなサイズのPLGA粒子で処理した後、両方の細胞形態が変化しなかったことは注目に値します(図4a、d、g、図5a、d、g、および図6a、 d、g)。 500nmおよび1µmのPLGA粒子は、唯一のUM-SCC-17A細胞(図5e、f、および図6e、f)で、唯一のTHP-1細胞(図5b、c、および図。6b、c)。共培養インキュベーション(図5h、i、および図6h、i)では、癌細胞で明らかなシグナルの減少が見られ、粒子の蛍光強度はマクロファージの蛍光強度よりも低いことがわかりました。マクロファージはすべてのタイプの粒子を迅速かつ効果的に貪食したため、癌細胞は共培養システムで十分な粒子を摂取できません。さまざまなサイズのPLGA粒子の癌細胞による取り込み能力に対する食作用の影響を定量化するために、半定量的な光学分析を実施しました。簡単に言えば、粒子蛍光強度は、例えば、50個の個々の粒子含有細胞に基づいて計算され、両方の細胞型の平均値を達成し、癌細胞の取り込み指数は、癌あたりの細胞内粒子蛍光強度を平均することによって決定された。マクロファージの細胞に正規化された細胞(図7)。単一培養では、100 nm PLGAの癌細胞取り込み指数は約1.34±0.19であることがわかりました。これは、癌細胞が単一細胞培養でマクロファージによって摂取された粒子よりも多くの粒子を摂取したことを示唆しています(図7)。 PLGA粒子の粘度が高く、喉頭がん細胞のサイズが大きいため、これは驚くべきことではありません。同様に、500nmと1µmのPLGAも、単作でそれぞれ約1.5±0.25と1.4±0.31の取り込み指数を持つ癌細胞によって大きく内在化されました。共培養システムにおけるマクロファージの存在下では、大きな粒子の取り込み指数は大幅に低下しました(0.59±0.12および0.47±0.1)。一方、混合細胞培養で100 nm PLGA粒子を24時間培養した後は、両方の細胞タイプで同一の細胞内在化機能が得られます。全体として、ここで蓄積された結果は、癌細胞およびマクロファージによる粒子の摂取が、単一細胞培養および共培養環境における取り込み経路の異なる経路に依存する可能性があることを示唆しました。 Moreover, the presence of macrophages reduced the cellular internalization of PLGA particles by cancer cells particularly for large ones, a biological event that has been observed in in vivo nanomedicine drug delivery studies [3, 15].
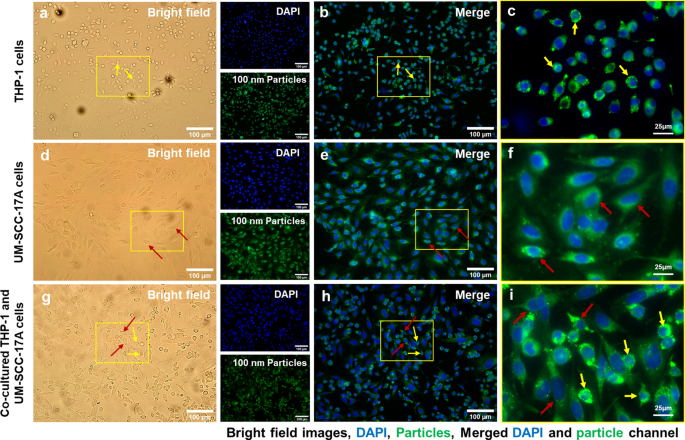
Microscopic examination of cellular internalization of 100 nm PLGA nanoparticles in monoculture and co-culture cells. Single type of cells or mixed cells was treated with 100 nm PLGA nanoparticles for 24 h at 37 °C at a concentration of 10 µg/mL. Cells were observed under bright-field (a 、 d 、 g ) and fluorescent channels (b, e, and h) with particles observed at the green channel and cell nuclei in the blue channel after stained with Hoechst. Massive cellular uptake of 100 nm PLGA nanoparticles can be visualized in magnified images (c 、 f 、 i ) for monoculture THP-1 macrophages (yellow arrows) and laryngeal cancer cells UM-SCC-17A (red arrows). In the co-culture system, 100 nm PLGA nanoparticles were still highly ingested by macrophages but less efficient for cancer cells compared to single cultured cells
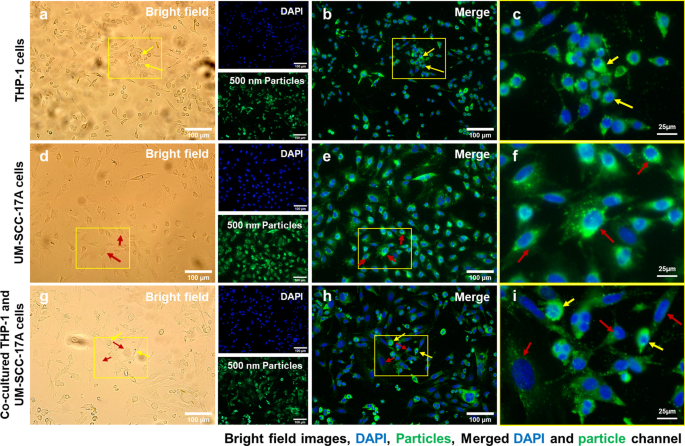
Microscopic examination of cellular internalization of 500 nm PLGA nanoparticles in monoculture and co-cultured cells. Single type of cells or mixed cells was treated with 500 nm PLGA nanoparticles for 24 h at 37 °C at a concentration of 10 µg/mL. Cells were observed under bright-field (a 、 d 、 g ) and fluorescent channels (b 、 e 、 h ) with particles observed at the green channel and cell nuclei in the blue channel after stained with Hoechst. Massive cellular ingestion of 500 nm PLGA nanoparticles can be visualized in magnified images (c 、 f 、 i ) for monoculture THP-1 macrophages (yellow arrows) and laryngeal cancer cells UM-SCC-17A cells (red arrows). In the co-culture system, 500 nm PLGA nanoparticles were still highly uptake by macrophages, while cancer cells had inadequate particle internalization
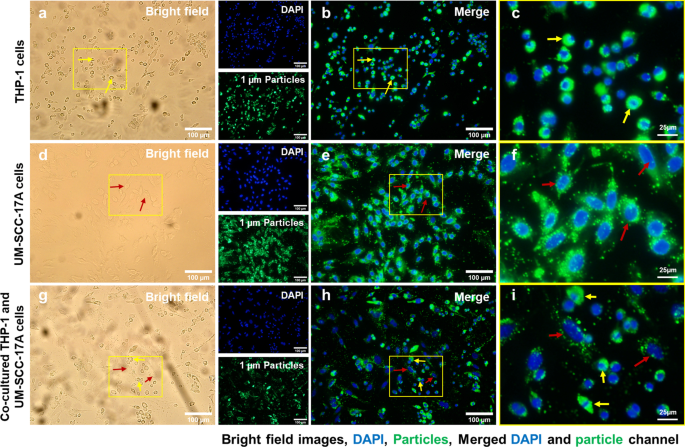
Microscopic examination of cellular internalization of 1 µm PLGA particles in monoculture and co-culture cells. Single type of cells or mixed cells was treated with 1 µm PLGA particles for 24 h at a concentration of 10 µg/mL. Cells were observed under bright-field (a 、 d 、 g ) and fluorescent channels (b 、 e 、 h ) with particles observed at the green channel and cell nuclei in the blue channel after stained with Hoechst. A large amount of particle uptake and tremendous accumulation of 1 µm PLGA particles can be visualized in magnified images (c 、 f 、 i ) for monoculture THP-1 (yellow arrows) and UM-SCC-17A laryngeal cancer cells (red arrows). In the co-culture system, 1 µm PLGA particles were still efficiently uptake by macrophages, while cancer cells had insufficient particle ingestion
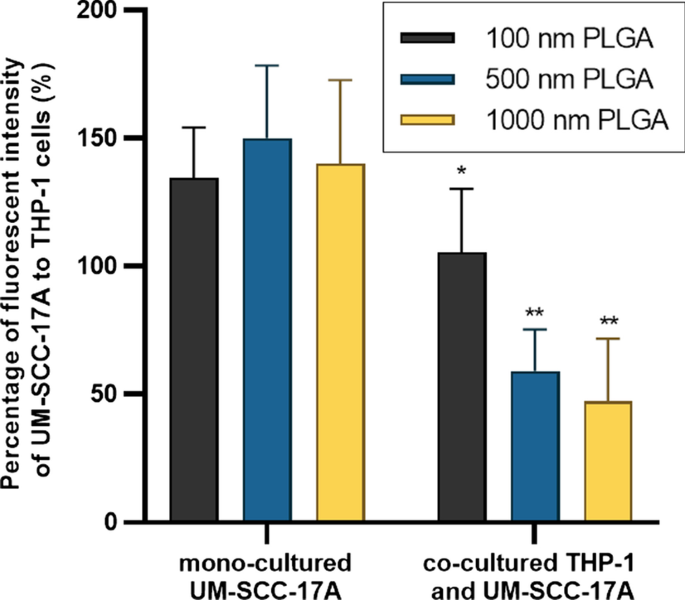
Quantitative analysis of PLGA particle internalization in UM-SCC-17A cells in monoculture and co-culture systems. The percentage of particle fluorescent intensity in UM-SCC-17A cells normalized to that in THP-1 cells for solely and mixed cultured cells
Quantification of cellular uptake by flow cytometry
Flow cytometry is widely used to determine of particle-cell interplay in a quantitative manner, for example, the size-dependent uptake of polystyrene particles with sizes ranging from 20 to 1000 nm by dendritic cells in vivo [35]. FACS was thus utilized for analysis of the percentage of NP-positive cells and particle number in those NP-positive cells in monoculture and co-culture systems. Then, average NP counts in a single cell were calculated by determining the total fluorescence intensity in particle-laden cells normalized to the fluorescence of total applied dose. For example, about 70% of 500 nm PLGA was deposited in the cancer cells, whereas the residual was kept in the supernatant of cell cultures (Additional file 1:S4). This fluorescence intensity measured by a Tecan reader was then compared to the average/total FACS signals (FITC signal values of P5 and P6 in Fig. 8,) to estimate the particle number in the co-culture system. As seeded in the co-cultured cells, about half of the cells were recognized as macrophages and the rest were considered as cancer cells. It was observed that approximately 13,000 of single 100 nm PLGA particles accumulated in cancer cells and 9700 particles in macrophages (Fig. 8i) in the monoculture, consistent with the above microscopic examinations. A much lower particle number (164 ± 30 and 45 ± 15) was ingested by monocultured cancer cells for 500 nm and 1 µm PLGA, with a slightly lower particle count in the respective macrophages (Fig. 8i). The number of particles ingested by single cells is in great agreement with the literature records [24]; for instance, an average 2500 of gold NPs coated with cetyltrimethylammonium bromide were deposited in epithelial cells, while PEG-modified gold NPs only had a few tens per cell [36]. In co-culture systems, unexpectedly, there is a slight increase of 100 nm particle number in cancer cells, despite a higher enhancement of NP internalization by macrophage. Comparing to the uptake index in the single-cell to mixed cell culture, it also reduces about 25% for 100 nm PLGA particles. The uptake indices were found to significantly decline with around 2.5 and 3 folds reduction in co-cultured system for 500 nm and 1 µm PLGA, respectively (Fig. 8i). Generally, it was found that the existence of macrophages largely affects the uptake ability of cancer cells especially for large particles, which is also in excellent agreement with the above observations.
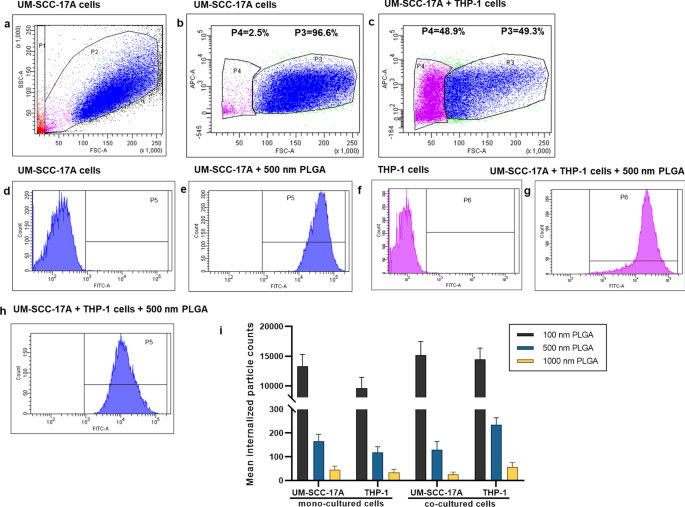
Particle uptake quantification in monoculture UM-SCC-17A cells and co-culture with THP-1 cells by flow cytometry. Grating strategy to identify respective cell populations in mixed cell culture (a – h )。 Gating is showing one representative experiment of cells exposure to 500 nm PLGA particles. With initial live gating in the y-axis with a side scatter (SSC-A) and x-axis with a forward scattering (FSC-A), P2 a were further gated with FSC-A versus APC-A to differentiate the THP-1 cells in P4 c from UM-SCC-17A cell population in P3 (monoculture cells (b ) and mixed cells (c ))。 The cell population of P3 further displayed as counts versus FITC plots (P5) in non-exposed cells (d ), monoculture cells (e ), and co-cultured cells (h )。 Also, P6 is the counts versus FITC-A plot originated from P4 population in non-exposed cells (g ) and co-cultured cells (f )。 Both types of cells efficiently ingested the 500 nm PLGA indicated by the solid histogram completed shifted to the right side of the x -axis, indicating particles are taken up by all exposed cells. Quantification of particle-laden numbers (i ) in both types of cells for mono-culture and co-culture systems
Currently, different uptake pathways by cancer cells in ingesting particles with different sizes and surface modifications like clathrin-mediated endocytosis, caveolae-mediated endocytosis, clathrin- and caveolae-independent endocytosis, micropinocytosis, and macropinocytosis have been claimed in the literature [37]. For instance, it has been proved that iron oxide aggregates with a size of < 200 nm are taken up by MCF-7 cells through the clathrin-mediated endocytosis, whereas larger aggregates tend to be ingested via macropinocytosis [38]. Another study has demonstrated that 100 nm plain polystyrene particles tended to be taken up mainly through macropinocytosis, whereas the internalization of carboxylated polystyrene particles prefer to occur via the clathrin-mediated endocytic route [25]. Phagocytosis is a classical uptake pathway for immune cells such as neutrophils, dendritic cells, and most importantly monocytes/macrophages. The uptake pathways tightly depend on various parameters of drug delivery vesicles, e.g. , the particle size, chemical composition, surface modification, proteins in the culture environment, as well as cell type. Usually, multiple uptake pathways can be involved in particle ingestion such as the caveolae-mediated endocytosis, clathrin-mediated endocytosis, and macropinocytosis, which has been found to participate in cellular internalization of 300–400 nm chitosan NPs in human HeLa cells [39]. It has also been observed that 63 nm cholesterol-modified pullulan NPs enter into the human hepatocellular carcinoma (HepG2) cells via macropinocytosis and clathrin-mediated endocytosis [40]. Several previous studies showed that PLGA particle cell uptake involves different endocytic pathways, in which clathrin-independent endocytosis is the main route responsible for the internalization of PLGA in in vitro models [23, 24, 37]. Nevertheless, once they entered into the cells, PLGA particles applied here were highly potentially entrapped by the endo-lysosomal system consisting of early endosomes, recycling endosomes, late endosomes, and lysosomes [41]. It should be noted, however, that there is a lack of studies showing the cellular uptake mechanisms in co- or tri-cultured systems in vitro, a notion that has to be probe in the future.
Induction of cell fusion by PLGA NPs
Extensive scientific evidence has shown that the fusion of monocytes/macrophages into multinucleated giant cells (MGCs) occurred in a broad range of biological processes [42, 43]. Generally, implantation of biomaterials into the body causes a foreign body response characterized by the fusion of macrophages into MGCs and fibrotic encapsulation [44]. A wide type of human and murine primary cells like alveolar macrophages, splenic macrophages, microglia, bone-marrow-derived macrophages, and blood monocytes, as well as cell lines such as RAW264.7 and UG3 were also frequently observed to form MGCs in vitro [26, 45]. It is well-known that the macrophages form a fusogenic phenotype when they are unable to ingest foreign materials via phagocytosis because of the large size of particles or implants. So far, it is unclear whether in vitro macrophage cell fusion relates to the particle size in the range 100–1000 nm, which can be easily phagocytized. Here, the cell fusions were observed in all groups by microscopic examination (Fig. 9). The cellular fusion was confirmed only when multiple nuclei were found to share the same cytoplasm in both fluorescence images and bright-field images. Spontaneous formation of giant cells by THP-1 cells was occurred in the control group without particle treatment (Fig. 9a), with a fusion percentage of about 10% of the total cells (Fig. 9h), as reported in the literature [46]. Size-dependent cellular fusion was found for 100 and 500 nm PLGA particles, whereas 1 µm PLGA-induced insignificant enhancement of fusions in monoculture. This may be due to the small number of 1 µm PLGA particles exposed to cells (approximately 60 particles exposed to each cell at a concentration of 10 µg/mL). When the macrophages were co-cultured with cancer cells, the percentages of MGC formation were increased in all groups in comparison with the corresponding monoculture groups. In particular, there is a significant increment of cellular fusion for 1 µm PLGA (19%) in the mixed cell culture. The most prominent increase in fusion occurred in co-cultured 500 nm PLGA particles, which might be attributed to the large size of particles and sufficient particle numbers ingested per cell.
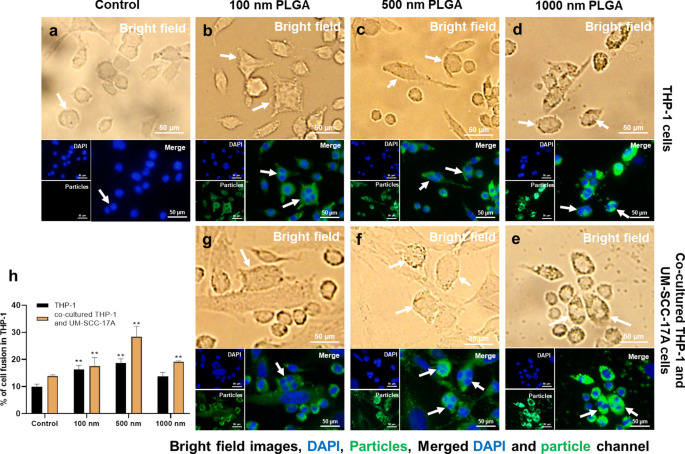
Visualization and quantification of THP-1 cell fusion in monoculture and co-culture systems. Cell fusion occurred in all groups including the control group without particle treatment for THP-1 cells (a )。 Monocultured (b 、 c ) 100 nm and 500 nm NP-induced significant cell fusion compared to 1 µm PLGA group (d ), which has a slightly higher level than that of the control group. In co-cultured systems, 100 nm (g ) and 1 µm (e ) PLGA had an identical percentage of cellular fusion, which is apparently smaller than that of 500 nm group (f )。 Quantification of cell fusion for all groups after 24 h incubation was displayed in h
The molecular machinery involved in macrophage fusion has been widely probed, achieving substantial progress [43]. For example, the formation of macrophage fusion receptors CD47and CD44 together allowed mediating the process of macrophage fusion, and subsequent the differentiation of giant cells [42] and miR-223 delivery by a NP vesicle permitted attenuating it [47]. Hence, further study should focus on the determination of key molecules in regulating particle-induced macrophage fusion and the dominant receptors expressed in the giant cell membrane. Another important concern is the fate of MGCs. It is believed that MGCs fused with particles or stimuli might subsequently experience the process of apoptosis or necrosis [48]. This may lead to the release of undigested particles or other giant materials to the other cells in vitro or biological tissues in vivo, further inducing the long-term inflammatory response or granulomas. On the other hand, the macrophage fusion itself may be benefit for NP drug delivery, for example, in tumorous tissues, the re-release of drugs from macrophages to the cancer cells might represent an enhanced tumor killing ability. More importantly, future nanomedicine study should address how to exploit the application of macrophage fusion for nanocarrier drug delivery to improved disease diagnosis and therapy. This is because not only macrophages an abundant type of immune cells in the body with the excellent uptake ability of nano-drugs, but also they may be used in targeted gene delivery for repair of injured tissues.
結論
To the best of our knowledge, this study first reported the competitive cellular uptake of PLGA particles with sizes ranging from 100 nm to 1 µm in co-cultured UM-SCC-17A laryngeal cancer cells and THP-1 monocytes/macrophages. The data collected here proved that immune cells may alter/lower the particle internalization by cancer cells in vitro, which is similar to the previous findings in in vivo nanocarrier drug delivery studies. Size-dependent and cell culture-related macrophage cellular fusion caused by PLGA particles has also been demonstrated here. Future studies should probe the uptake mechanism in co-cultured systems and design novel approaches to achieve a higher uptake index for laryngeal cancer cells in the presence of phagocytes. Moreover, elaborate evaluation of intracellular trafficking and fate of nano-drug-carriers in co- or tri-cultured systems in 3D, which better mimic the in vivo conditions, is needed prior to the utilization of animal studies in vivo and even in clinical trials.
データと資料の可用性
この調査中に生成または分析されたすべてのデータは、この公開された記事[およびその補足情報ファイル]に含まれています。
略語
- PLGA:
-
Poly(lactic-co-glycolic acid)
- HNSCC:
-
Head and neck squamous cell carcinoma
- EPR:
-
Enhanced permeability and retention
- NP:
-
ナノ粒子
- ICG:
-
Indocyanine green
- MPS:
-
Mononuclear phagocyte system
- LDH:
-
乳酸デヒドロゲナーゼ
- FACS:
-
Fluorescence-activated cell sorting
- MGC:
-
Multinucleated giant cells
- DLS:
-
動的光散乱
ナノマテリアル
- ドラッグデリバリーを強化するためのナノファイバーとフィラメント
- 卵巣癌細胞の高感度かつ迅速な検出のための柔軟なグラフェンベースのバイオセンサーのデモンストレーション
- 癌治療のためのナノ粒子:現在の進歩と課題
- 生体適合性FePO4ナノ粒子:ドラッグデリバリー、RNA安定化、および機能的活性
- 癌用途のための細胞ベースのドラッグデリバリー
- 黒色腫の標的化学療法治療のための薬物送達担体としての131I追跡PLGA-脂質ナノ粒子
- 子宮頸がんにおける抗がん効果を高めるための葉酸受容体を標的としたバイオフラボノイドゲニステインをロードしたキトサンナノ粒子
- 強化された細胞内在化のためのFe3O4磁性ナノ粒子の葉酸とgH625ペプチドベースの機能化の比較
- トランスフェリン受容体による細胞内在化が増強されたパクリタキセルベースの標的脂質ナノ粒子の抗増殖およびアポトーシス誘発能—白血病細胞での研究
- 強化された光触媒評価と抗菌分析のためのZnOナノ粒子に対するMgドーピングの影響
- 膀胱癌細胞を阻害するための疎水性修飾プルランナノ粒子を用いたミトキサントロンの新規送達および阻害効率に対するナノ薬物サイズの影響