感染性微生物の効率的な二光子励起光線力学的不活性化のためのヒドロキシル基依存性を有する水溶性フラレノール
要約
水溶性フラレノール[C 60 (OH) 46 ]高い一重項酸素量子収率を示し、効率的に活性酸素種を生成しました。さらに、水溶性C 60 (OH) 46 露出したヒドロキシル基のより高い組成を有するものは、そのような基のより低い組成を有するものと比較して、優れた二光子安定性および特性を有していた。したがって、調製されたフラレノールは、効果的な二光子光増感剤となり得る。水溶性C 60 (OH) 46 良好な二光子特性を持っていた。二光子光線力学療法中、水溶性C 60 (OH) 46 Escherichia coli に対して実質的な抗菌活性を示しました 211.2nJピクセル -1 の超低エネルギーレベルで 800回のスキャンと760nmの光励起波長で。
はじめに
過去数十年にわたって、さまざまな光増感剤(PS)分子が合成されてきました[1]。ただし、既存のPSの臨床応用にはいくつかの問題があります。ほとんどのPS分子は疎水性であり、水性媒体中で容易に凝集するため、量子収率(QY)が低下します[2]。さらに、凝集したPSを単純に静脈内注射することはできません。健康な細胞への損傷を防ぐために、死んだ組織にPS分子を選択的に蓄積することも必要です。これらの問題のために、効果的なPSキャリアの開発は、光線力学療法(PDT)の主要な課題であり続けています。したがって、PSキャリアとしてナノ粒子を使用することへの関心が高まっています。
ナノバイオテクノロジーの進歩により、炭素原子、すなわちフラーレンC60のみで構成される新しいクラスのナノ構造[3,4,5,6,7,8,9,10,11]の生物医学的応用への関心が高まっています。 、無毒で独特の物理化学的性質を持つ球状分子(直径0.72 nm)です。親油性C 60 の小さいサイズ 分子は、生体分子との立体的適合性に関与し、膜の疎水性領域への統合を促進します[12、13]。拡張されたπのため -分子軌道の共役系、フラーレンC 60 紫外可視(UV-vis)光を吸収し、ほぼ100%の一重項酸素QY(Φ)で活性酸素種(ROS)を生成できます。 Δ )。さらに、フラーレンC 60 の物理化学的性質 ROSを生成し、PDTのPSとして機能できるようにします。フラーレンはまた、酸化促進効果を誘発する可能性があり、これは、使用されるフラーレン、調査された細胞型、および実験設定によって決定される可能性があります[14、15、16、17]。 C 60 極性溶液への溶解度が非常に低いため、医療への応用が大幅に制限されます。ただし、二重結合が存在するため、C 60 化学基を使用して簡単に変更し、水溶性を高めることができます。したがって、水溶性C 60 派生物は、神経保護、薬物および遺伝子送達、光増感、およびバイオセンシングを含む医療用途の機会を増やしています。
多光子レーザー顕微鏡(2光子レーザー顕微鏡とも呼ばれます)では、局所的な「非線形」励起を使用して、薄いラスタースキャン平面内でのみ蛍光を励起します。二光子レーザー顕微鏡は、さまざまな画像研究で使用されてきました[18]。これは通常、近赤外線(NIR)レーザー励起と組み合わせて、バイオイメージングに固有の最大組織透過率を利用します。これは、NIRには、わずかな散乱、低エネルギー吸収、最適な照射透過、および試料の光退色の低減という利点があるためです。 2光子レーザー顕微鏡とNIRレーザー励起の組み合わせは、厚い組織やより深い生体試料の蛍光顕微鏡に適した手法になり[19、20]、他の光励起療法にも広く適用されています[21、22]。さらに、その超低エネルギーと短い光励起のために、2光子レーザー顕微鏡はPDTを実行するための代替アプローチと考えられています[23]。一部のPSは毒性がありますが[24、25]、Φが高いPSは Δ PDTの実施が優先されます。高いΦ Δ 2光子技術を使用して光特性の分子活性を評価し、非線形顕微鏡研究を効率的に実施する場合、この値は特に望ましいものです。試料への入力エネルギー束に吸収されるエネルギーの比率が高く、試料への光損傷の可能性を最小限に抑えるため、このような値が望ましい[26]。ただし、文献には、PDT用の2光子特性を持つ材料の使用を検討した研究は含まれていません。この研究のギャップを埋めるために、本研究では、強力な電子供与能力と大きなπを備えた水溶性ヒドロキシル化フラレノールを適用しました。 -共役系により電荷移動効率が向上し、2光子特性が向上します。具体的には、水溶性ヒドロキシル化C 60 (OH) 46 は、超低エネルギーフェムト秒レーザー照射と2光子励起(TPE;励起波長760 nm)下でのわずか800スキャンを使用して、効果的な微生物除去のための2光子PSとして導出および適用されました。超低エネルギーフェムト秒レーザー照射の場合、エネルギーは211.2nJピクセル -1 でした。 出力は2.112mWでした(対物レンズ後のレーザー出力の計算については、「材料と方法」のセクションと図1aを参照してください。 x – y 焦点と z -レーザーシステムの軸分解能は、それぞれ約0.37538および0.90159μmです。さらに、スキャンプロセスの場合、合計有効露光時間は約3.2621 s、スキャンレートは4.0776 ms scan -1 でした。 、スキャン領域は200×200μm 2 (計算の詳細については、「材料と方法」のセクションを参照してください)。水溶性ヒドロキシル化C 60 (OH) 46 Escherichia coli のほぼ100%の除去を達成しました ( E.coli 、グラム陰性菌株)。さらに、水溶性C 60 (OH) 46 ヒドロキシル基の組成が高い場合、TPE下でのヒドロキシル基の組成が低い場合と比較して、優れた2光子光特性を示しました。したがって、誘導された水溶性C 60 (OH) 46 悪性微生物を排除するために同時PDTで使用する可能性がかなりあると見なすことができます。
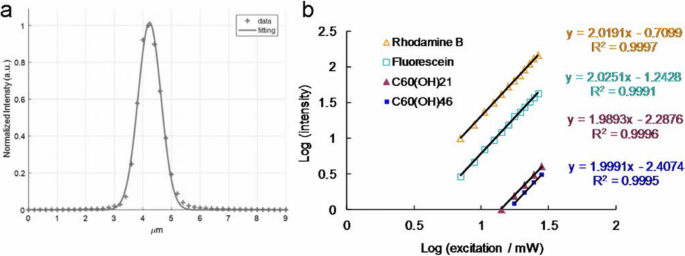
a z によると -さまざまな位置での第二高調波発生の信号を測定するために使用される金の薄膜の軸スキャン、 z -レーザーシステムの軸分解能(半値全幅)は約0.90159μm(ガウス関数を使用したフィッティング)です。 b 材料およびフルオロフォアの励起パワー(対数)に対するTPL強度の依存性。 704.0から2816.0nJピクセル -1 までのTPE露出 ローダミンBおよびフルオレセインの場合、1408.0〜2816.0nJピクセル -1 水溶性C 60 の場合 (OH) 21 フレレノール、および1760.0〜2816.0 nJ pixel -1 水溶性C 60 の場合 (OH) 46 フレレノール。励起波長、760nm。投与量:OD 600 Eの0.05。コリ および3μgmL -1 材料。データは平均値±SD( n =6)
材料と方法
水溶性フラレノールの調製と特性評価、C 60 (OH) 21 およびC 60 (OH) 46 [27]
生のフラーレンは商業的に入手され(Sigma-Aldrich、セントルイス、ミズーリ州、米国)、C 60 (OH) 12 前述のように、前駆体が生成された。最初に、30%過酸化水素溶液(100 mL; Sigma-Aldrich、セントルイス、ミズーリ州、米国)を出発物質である0.5〜1.0gのC 60 に添加しました。 (OH) 12 、混合物を空気中60℃で激しく撹拌した。冷却後、2-プロパノール、ジエチルエーテル、およびヘキサンを含む溶媒の混合物(それぞれ100〜200 mL、Sigma-Aldrich、ミズーリ州セントルイス、米国)を溶液に加え、続いて遠心分離してデカントしました。残りの固体を、遠心分離およびデカンテーション手順により、200mLのジエチルエーテルで2回洗浄した。最後に、水溶性C 60 の最終製品 (OH) 21 およびC 60 (OH) 46 残留物をそれぞれ室温で一晩真空下で乾燥させることにより得られた。最終製品の重量は、熱重量分析によって較正されました。最終製品の形態は、高分解能透過型電子顕微鏡(HR-TEM、JEOL 3010、昭島、東京、日本)を使用して、C 60で約1.11±0.03nmおよび1.13±0.04nmの分解能で観察されました。 (OH) 21 およびC 60 (OH) 46 、 それぞれ。動的光散乱法(DLS、Malvern Nano-ZS90、ウスターシャー、ウェストミッドランズ、英国)も、材料のサイズを決定するために使用されました。調製されたままの材料の露出された官能基は、最初にフーリエ変換赤外(FTIR)分光法(RX1、PerkinElmer、マサチューセッツ州ウォルサム、米国)によって調べられました。材料のUV-vis分光法は、分光計(U-4100、日立、千代田区、東京、日本)を使用して実施しました。フレレノールの表面化学は、X線光電子分光法(XPS、PHI 5000分光計(VersaProbe、Chanhassen、MN、USA))によって調べられました。フレレノールの分子量は、電界脱離(FD)質量分析計(AccuTOF、GCx-plus、JEOL、昭島、東京、日本)を使用して決定され、ヒドロキシル基の数は、結果に基づいて21および46であることが確認されました。それぞれ。
細菌培養[28]
E。コリ 、私たちの研究室から得られた、LBの栄養寒天培地(1リットルあたり:トリプトン10 g、酵母エキス5 g、塩化ナトリウム8 g、寒天15 g、pHを7.5に調整)(Sigma-Aldrich、セントルイス、 MO、USA)および37°Cでインキュベート。
コロニー形成単位(CFU)カウント法による生体適合性アッセイ[28]
E。コリ (OD 600 〜0.05)に材料(0–9μgmL -1 )を加えました )、37°Cで3時間インキュベートします(追加ファイル1:図S1)。インキュベーション後、混合物を遠心分離し、細菌のペレットを希釈しました(OD 600 〜0.05)。 10 -5 の希釈係数 〜10 -8 次に、培養された細菌で実施され、寒天プレート上にプレーティングされた。プレートはインキュベーター(37°C)に一晩置きます。生き残った細菌の数が決定され、CFU mL -1 の単位に対応するパーセンテージ(%)として表されました。 インキュベーション後。データは平均値±SD( n =6)。
ψ Δ 測定[29、30]
以前の研究によると、ψ Δ 取得できる。 ψ Δ 測定はD 2 で実行されました meso を使用して、355nmでO -参照としてのテトラ(4-スルホナトフェニル)ポルフィン二塩酸塩(TSPP; Sigma-Aldrich、セントルイス、ミズーリ州、米国)(ψ Δ =0.64)。
蛍光QY測定[31、32]
造影剤の相対フォトルミネッセンス(PL)QYは通常、放出された光子と吸収された光子の比率であり、次のように与えられます。
$$ \ mathrm {QY} ={\ mathrm {QY}} _ {\ mathrm {ref}} \ \ left({\ eta} ^ 2 / {\ eta _ {\ mathrm {ref}}} ^ 2 \ right) \ left(I / A \ right)\ left({A} _ {\ mathrm {ref}} / {I} _ {\ mathrm {ref}} \ right)$$(1)ここで、QY ref =0.28は、参照としてジメチルスルホキシド(DMSO; Sigma-Aldrich、米国ミズーリ州セントルイス)に溶解したCy5.5のQYです。η はddH 2 の屈折率です O =1.33(η ref DMSOの=1.48)、 I は統合された蛍光強度と A は励起波長での吸光度です。一光子励起(OPE)またはTPEは同じQYを生成します。
2光子吸収(TPA)および2光子発光(TPL)を測定するためのフェムト秒レーザー光学システム[23、28、33、34、35 、36,37,38]
以前の研究によれば、自家製のフェムト秒チタンサファイア(ti-sa)レーザー光学システム(繰り返し率80 MHz; Tsunami、Spectra-Physics、Santa Clara、CA、USA)が使用されました。
TPA測定
検流計スキャナーの速度は2m ms -1 、励起スペクトルは、励起パワー2.8 mWで720〜820 nmと測定されました[これは、対物レンズ前のパワーです。対物レンズ後(またはサンプル上)のパワーは0.9856mWまたは98.56nJピクセル -1 ]。したがって、フラレノールの励起波長の関数としての相対TPAスペクトルを測定しました。
TPLスペクトルの測定
材料は、励起波長760 nm、スキャン領域200×200μm 2 でフェムト秒レーザーからのTPEにさらされました。 、周波数10 kHz、露光時間1.638 s /(スキャン、ピクセル)=100μs、128×128ピクセルスキャン -1 、および1562.5×1562.5 nm 2 のピクセル領域 。焦点面積はπ d として計算されました 2 / 4、ここで d =0.61λ/開口数( NA )は、ビームウエストの半値全幅です。たとえば、 x で – y 760nm励起の軸焦点と NA の×40油浸対物レンズ 1.3の d =0.61×800nm / 1.3 =375.38 nm =0.37538μm、および z -軸分解能は0.90159μmと測定されました。 760 nm励起の場合、個々のナノ材料のスキャンあたりの露光時間は、(焦点スポット面積/ピクセル面積)×100 =4.0776 ms、および総露光時間 t として表されます。 =4.0776ms×スキャン数。 ×40の油浸対物レンズ( NA 1.3)を使用して信号を収集し、スペクトル光度計の検出範囲は300〜695nmでした。
さらに、レーザー出力の計算(mWまたはnJ pixel -1 )サンプルに使用したものは次のとおりです。 ×40の油浸対物レンズ( NA 1.3)、この光学システムでは、波長760 nmでの透過率は約88%であり、出力から対物レンズへのレーザー出力は、出力の損失により元の出力出力の40%にすぎません。結果として、(サンプル上の)目的の後の計算されたエネルギーは P です。 出力 (mW)* 40%* 88%=0.352× P 出力 (mW)。たとえば、 P 出力 =2.8 mW、(サンプル上の)対物レンズ後の計算されたエネルギーは3.0 mW * 40%* 88%=0.9856mWです。スキャンレートが10kHzの場合(各パルスは0.1msピクセル -1 のままです )、サンプルで計算されたエネルギー(J pixel -1 )は P あたりでした 出力 (mW)* 40%* 88%* 0.1 ms =0.0352 * P 出力 (J pixel -1 )。たとえば、 P 出力 =2.8 mW、エネルギー(J pixel -1 )サンプル上=2.8 mW * 40%* 88%* 0.1 ms =0.09856μJpixel -1 =98.56nJピクセル -1 。目標(サンプル上)の後の電力が使用され、この原稿のスループットがマークされました。
TPE絶対断面積の測定[24、36、37、38、39、40、41、42、43、44、45、46、47、 48]
TPEの絶対断面積は、経由で発光信号を測定しました。 以前の研究によるフェムト秒レーザー光学システム。フルオレセインとローダミンB(Sigma-Aldrich、セントルイス、ミズーリ州、米国)のTPLを検証する必要がありました。結果を図1bに示し、704 nJ pixel -1 の励起パワー範囲で発光強度の依存性を測定することによって得られました。 (7.04 mW)から2816nJピクセル -1 (28.16 mW)。フルオレセインの指数が2.03、ローダミンBの指数が2.02の二次依存性を測定して、TPEからの発光を測定するための励起パワーを増加させました。以前の研究によると、フルオレセインとローダミンBのTPEの作用断面積は36.4と68.0 GM(1 GM =10 -50 )です。 cm 4 sフォトン -1 )、それぞれ、760nm励起の場合。また、Chris Xu教授(コーネル大学、ニューヨーク州、米国)から提供された無料のWebサイトhttp://www.drbio.cornell.edu/cross_sections.htmlも参照しました。フルオレセインとローダミンBのTPE作用断面積は、それぞれ36.5と66.1 GMと計算され(表1)、徐教授の研究室のものと比較して5%未満の誤差を示しました。この研究では、断面積を決定するための標準参照としてローダミンBを選択し、水溶性C 60 のTPEの絶対断面積を計算しました。 (OH) 21 およびC 60 (OH) 46 フレレノールはそれぞれ約1230.51GMと1037.21GMでした。サンプルのTPE絶対断面積を計算するために測定されたパラメーターを表3に示します。2光子特性と2光子光力学的能力の材料でバッチ間の変動は観察されませんでした。
<図> 図>フェムト秒レーザー光学システム(蛍光寿命イメージング顕微鏡、FLIM用)[39、45]
以前の研究によれば、自家製のフェムト秒ti-saレーザー光学システム(繰り返し率80 MHz; Tsunami、Spectra-Physics、Santa Clara、CA、USA)が使用されました。寿命データとパラメータは、TPE(Ex、760 nm)での発光を監視しながら、三重指数方程式フィッティングを使用して生成されます。
放射性および非放射性崩壊率の計算[46]
PL QYと寿命は、さまざまな環境での蛍光色素の発光特性を調査する際の主要なパラメーターです。 QY( Q )は次のように表すことができます:
$$ Q =\ frac {\ varGamma} {\ varGamma + k} $$(2)ここで、Γ は放射性崩壊率であり、 k 非放射性崩壊率です。蛍光寿命は通常、励起状態の電子が基底状態に崩壊するのに必要な平均時間として定義されます。 TPLの寿命τ 減衰率に関連することもあり、次のように説明されます。
$$ \ tau =\ frac {1} {\ varGamma + k} $$(3)次の式。 (2)と(3)では、放射性と非放射性の減衰率を計算できます。
光子を吸収すると、蛍光分子の弱く結合した電子の1つであるフルオロフォアがより高いエネルギーレベルに昇格します。その後、フルオロフォアは励起状態になります。 A * 。この状態は準安定です。したがって、フルオロフォアは安定した基底状態 A に戻ります。 。これは、蛍光光子hνを放出することによって放射的に行うことができます。
$$ A \ ast-> A + h \ nu $$または、励起状態のエネルギーを熱として放散することにより、非放射的に:
$$ A \ ast-> A + \ mathrm {heat} $$励起状態の過疎化は、利用可能な脱励起経路に依存します。蛍光は、最初の電子的に励起された一重項状態 S の最低振動エネルギーレベルの放射不活性化です。 1 、電子基底状態に戻る、 S 0 。一重項状態は、スピンフリップなしで弱く結合した電子が占めることができるエネルギー準位です。吸収と放出のプロセスは、アレクサンダー・ヤブロンスキーにちなんで名付けられたエネルギー準位図によって示されています。
蛍光寿命、τ 、は、フルオロフォアが電子的に励起された状態に留まる平均時間です S 1 励起後。 τ は、すべての励起状態の過疎化プロセスのレートパラメータの合計の逆数として定義されます。 (3)、ここで非放射速度定数 k 内部転換の速度定数の合計 k ic 項間交差の三重項状態 k への速度定数 isc k のように = k ic + k isc 。蛍光発光は常に S の最低振動レベルから発生します 1 、カシャの法則として知られる規則。フルオロフォアにはその励起経路の記憶がないことを示します。たとえば、OPEとTPEは同じ蛍光スペクトル、QY、および寿命をもたらします。
レーザー照射後の細菌生存率の決定[28]
CFUカウント方法
バクテリア(OD 600 〜0.05)に材料(3または6μgmL -1 )を加えました )暗所で37℃で3時間インキュベートする。インキュベーション後、混合物を遠心分離し、細菌のペレットを希釈しました(OD 600 〜0.05)、211.2nJピクセル -1 のTPEパワーにさらされます 800スキャン(総有効露光時間の約3.2621 s;例:760 nm)。次に、10 -5 の希釈係数 〜10 -8 次に、培養された細菌で実施され、寒天プレート上にプレーティングされた。プレートはインキュベーター(37°C)に一晩置きます。生き残った細菌の数が決定され、CFU mL -1 の単位に対応するパーセンテージ(%)として表されました。 インキュベーション後。データは平均値±SD( n =6)。
LIVE / DEADキット
バクテリア(OD 600 〜0.05)に材料(3または6μgmL -1 )を加えました )暗所で37℃で3時間インキュベートする。インキュベーション後、混合物を遠心分離し、細菌のペレットを希釈しました(OD 600 〜0.05)、211.2nJピクセル -1 のTPEパワーにさらされます 800スキャン(総有効露光時間の約3.2621 s;例:760 nm)。次に、LIVE(SYTO 9、緑色蛍光で表示)/ DEAD(ヨウ化プロピジウム)を使用してペレットを染色しました。 指示に従って、PI、赤色蛍光で表示)キット(Thermo Fisher Scientific、米国マサチューセッツ州ウォルサム)。バクテリアの生存率は抗菌テストのために定量化され、ナノマテリアルで処理されたバクテリアのほぼすべてが処理後に死んでいることが示されました。同様の生存率は、PDTの材料の効率的な抗菌効果を決定するためにCFUカウント法によって定量化されました。データは平均±SD( n )として表されます =6)。
ROS検出[23、29、34、35、49、50、51、52、53、54、55]
一重項酸素( 1 O 2 )
(a)材料(3または6μgmL -1 )バクテリア(OD 600 〜0.05)、その後、暗所で37℃で3時間のインキュベーションに供した。続いて、混合物をTPE光励起(211.2 nJ pixel -1 )にさらしました。 、800スキャン; Ex、760 nm)、最後に一重項酸素センサーグリーン(SOSG)試薬(1μM; Thermo Fisher Scientific、米国マサチューセッツ州ウォルサム)と混合(Ex / Em:488/525 nm)。測定には蛍光分光計を使用した。 ROSを中和するために、混合物を30ppmの酸化防止剤αと混合しました。 -暗闇の中でトコフェロール/リノール酸メチル(Sigma-Aldrich、セントルイス、ミズーリ州、米国)を使用し、同じ処理でTPE光励起にさらしました。 (b)材料(3または6μgmL -1 )バクテリア(OD 600 〜0.05)、その後、暗所で37℃で3時間のインキュベーションに供した。続いて、混合物をTPE光励起(211.2 nJ pixel -1 )にさらしました。 、800スキャン;例、760 nm)、最後に10μMのトランス-1-(2'-メトキシビニル)ピレン( t -MVP、Thermo Fisher Scientific、米国マサチューセッツ州ウォルサム)/0.10 M SDS(Sigma-Aldrich、米国ミズーリ州セントルイス)(Ex / Em:352/465 nm)。 ROSを中和するために、混合物を30ppmの酸化防止剤αと混合しました。 -暗闇の中でのトコフェロール/リノール酸メチル(Sigma-Aldrich、セントルイス、ミズーリ州、米国)。 t の反応 - 1 のMVP O 2 1-ピレンカルボキサルデヒドに分解する間に蛍光を発するジオキセタン中間体を生成します。さらに、この選択性の高い蛍光プローブは、ヒドロキシルラジカル、スーパーオキシド、過酸化水素などの他の活性酸素種とは反応しません。測定には蛍光分光計を使用した。 ROS中和は、前述の処理と同じ方法で実施しました。
スーパーオキシドラジカルアニオン(O 2 .− )
(a)材料(3または6μgmL -1 )バクテリア(OD 600 〜0.05)、その後、暗所で37℃で3時間のインキュベーションに供した。続いて、混合物をTPE光励起(211.2 nJ pixel -1 )にさらしました。 、800スキャン; Ex、760 nm)そして最後に2,3-ビス(2-メトキシ-4-ニトロ-5-スルホフェニル)-2H-テトラゾリウム-5-カルボキサニリド(XTT、0.45 mM; Sigma-Aldrich、ミズーリ州セントルイス)と混合、 米国)。この資料の目的は、O 2 と相互作用することでした。 。 − XTT-ホルマザンを生成し、強い吸収(波長470 nm)をもたらしました。この吸収を監視するために、UV-vis分光計が採用されました。 ROSを中和するために、混合物を30ppmの酸化防止剤αと混合しました。 -暗闇の中でトコフェロール/リノール酸メチル(Sigma-Aldrich、セントルイス、ミズーリ州、米国)を使用し、同じ処理でTPE光励起にさらしました。 (b)材料(3または6μgmL -1 )バクテリア(OD 600 〜0.05)、その後、暗所で37℃で3時間のインキュベーションに供した。続いて、混合物をTPE光励起(211.2 nJ pixel -1 )にさらしました。 、800スキャン;例、760 nm)、最後に50 mM重炭酸緩衝液(pH 8.60)およびグルタチオン(γ)と混合します。 -l-グルタミル-1-システイニル-グリシン、GSH、Sigma-Aldrich、セントルイス、ミズーリ州、米国)/0.80 mM重炭酸塩バッファー(O 2 のエルマンアッセイ 。 − 検出)。続いて、以前の研究の手順に従って、以下の実験を行った。 GSHの損失(%)は、サンプルとネガティブコントロール間の吸光度の差をネガティブコントロールの吸光度で割ったものとして計算されました。生成されたO 2 の信号 。 − 前の計算で説明したように取得されました。データは平均値±SD( n =6)。
取り込みアッセイ[35]
E。コリ (OD 600 〜0.05)を3μgmL -1 とインキュベートしました 材料。 3μgmL -1 の量の吸光度 材料はUV-vis分光法(Abs、約203 nm)で記録されました。材料は Eと混合された。コリ (OD 600 それぞれ37°Cで1時間目から10時間目まで〜0.05)、遠心分離(1200 rpm)して余分な物質を除去し、上清を保持してその吸光度を測定します。収集した上澄みと元の材料との間の吸光度の差を推定し、各時点での取り込みのパーセンテージをもたらした。データは平均値±SD( n =6)。
統計分析[56]
統計的有意性は分散分析によるものでした。 p 値は、すべての処理で統計的に有意であると見なされました。
結果と考察
水溶性フラレノールの特性評価
水溶性C 60 (OH) 46 環状で単分散であると決定された(フラレノール)は、以前の研究[27]に従って合成された。低倍率(図2a)およびHR-TEM画像(図2b)を使用して決定した場合、フラレノールの平均横方向サイズは約1.13±0.04nmでした。さらに、フラレノールは、 d に対応する良好な格子間隔とともに、良好な結晶化度を示すことが認められました。 -フラレノール{1 \(\ overline {1} \)00}格子縞の間隔。ただし、これらの粒子は、pH7.0の水溶液中で水素結合を介して凝集体を形成する可能性があります。 DLS分析で明らかになったように、形成された凝集体の平均サイズは約130nmでした。さらに、凝集体は、pH 7.0水溶液、1×リン酸緩衝生理食塩水、培地などのさまざまな生理学的環境で3か月間非常に安定したままでした(追加ファイル1:表S1)。フレレノールのUV-vis吸収スペクトルでは、約216および309 nmに吸光度のピークが観察され、これらのピークはπに起因していました。 – π *芳香族C =C結合と n の遷移 – π *それぞれC =Oショルダーの遷移。 π -水性分散液で通常観察されるように、フラレノールの電子遷移には酸素が含まれており(図2c)、それによってフラレノールの存在が確認されました。 FTIR、XPS、および質量分析を使用して追加の特性評価を行い、調製した材料の特性を確認しました。 FTIRを使用して、調製した材料の露出した官能基を分析しました。分析結果から、次の特徴的な材料バンドが明らかになりました。約1109 cm -1 のC–O伸縮バンド (バンド1)、約1271 cm -1 のフェノール性C–OH伸縮バンド (バンド2)、約1422 cm -1 の第三級アルコールC =O伸縮バンド (バンド3)、約1674 cm -1 のC =Cストレッチバンド (バンド4)、約1721 cm -1 のC =O伸縮バンド (バンド5)、および約3318 cm -1 のC–H分子間水素結合およびカルボン酸塩O–H伸縮バンド (バンド6)。さらに、CO 2 のバンド 干渉が観察されました。これらのバンドは、露出したヒドロキシル基とカルボニル基、および芳香族C =C結合を示しました(図2d)。 XPSは、一般に炭素原子を主に含むフラレノールの表面化学を調べるために実行されました。フレレノールのデコンボリューションされたC(1s)スペクトルは、非酸素化環(C–C / C =C、286.1 eV)、C–O結合(286.9 eV)、およびC =O結合(288.0 eV)を示しました。また、O(1s)/ C(1s)比は約35.8%でした(図2e)。フレレノールの分子量は、FD質量分析を使用して決定されました(追加ファイル1:図S2)。 the number of hydroxyl groups (C–OH) was confirmed to be 46, which was consistent with the atomic ratios and bonding compositions of the fullerenol summarized in Fig. 2. These characterization results confirm the successful synthesis of fullerenol.
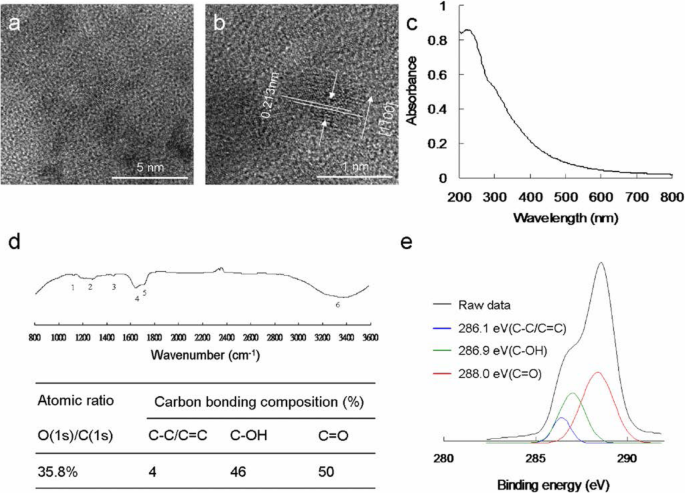
Functional characterization of the synthesized water-soluble C60 (OH)46 fullerenol. a Low-magnified TEM image and b HR-TEM image of a water-soluble fullerenol illustrating the materials {1\( \overline{1} \)00} lattice planes and the mean size of 1.11 ± 0.03 nm with a d -spacing of 0.213 nm. c UV–vis and d FTIR spectra of nanomaterial. e Deconvoluted C(1s) XPS spectra and fitted peaks obtained using Gaussian function:nonoxygenated ring (C–C/C=C), C–O bond, and C=O bond, respectively. The atomic ratio and bonding composition of fullerenol are shown as summarized in the table. The O(1s)/C(1s) atomic ratio is 35.8%
ROS Generation of Water-Soluble Fullerenol Under TPE
A PS absorbs and transfers light energy to other nonabsorbing molecules to generate ROS, which kill targeted cells, damage tumor vasculature, and activate an antitumor immune response. PSs have a particular arrangement of electrons in their molecular orbitals. Similar to nearly all molecules, at ground (singlet) state, PSs have couples of electrons with opposite spins in low-energy molecular orbitals. The absorption of light at an appropriate wavelength lifts an electron to a high-energy orbital without changing its spin. This is a short-lived (nanoseconds) excited singlet (S1 ) state, and the PS can lose its energy and return to the ground state by emitting light (fluorescence) or heat. Alternatively, intersystem crossing, wherein the spin of the excited electron is inverted, can occur in the S1 州。 This electron spin inversion is responsible for the relatively long life (lasting microseconds) of the excited triplet (T1 ) state. Radiative triplet-to-singlet transitions are inhibited because they require a change in electron spin, which is a slow process. From the T1 state, the PS can return to the ground state by emitting light (phosphorescence) or transferring energy to another molecule. It can also lose energy through internal conversion or radiationless transitions when colliding with other molecules. The longer the life of the PS in the T1 state is, the higher are its chances of colliding with another molecule, resulting in ROS production [57,58,59]. The photosensitization of water-soluble fullerenols results in their transition to a long-lived T1 state and subsequent energy or electron transfer to molecular oxygen, yielding ROS such as 1 O 2 およびO 2 。 − , which have major roles in PDT. Therefore, 1 O 2 およびO 2 。 − produced by water-soluble C60 (OH)46 must be detected directly using laser irradiation. To detect 1 O 2 およびO 2 。 − formation during PDT, in this study, PDT was initiated by combining excited the triplet water-soluble C60 (OH)46 , oxygen, and light configured to a suitable wavelength and energy as well as by introducing SOSG, t -MVP, XTT, and GSH reagents [33, 34, 49,50,51]. To exploit the potential bactericidal capability of the materials, a wavelength of approximately 760 nm was determined to be the most efficient for deriving the relative maximum TPA ratio of the water-soluble C60 (OH)46 under TPE (Fig. 3a); this is attributable to the interband transitions involved [52]. This wavelength was used in subsequent experiments in this study. The water-soluble C60 (OH)46 was photoexcited through TPE at a power of 211.2 nJ pixel −1 with 800 scans (Ex, 760 nm; total effective exposure time, ~ 3.2621 s) and delivered dose of 3 or 6 μg mL −1 (Additional file 1:Table S2). Furthermore, to confirm the involvement of ROS in the PDT effects of the water-soluble C60 (OH)46 、α -tocopherol was used for ROS neutralization [49, 53]. The quantity of generated ROS was reduced after the addition of α -tocopherol, but the observed bacterial viability increased as expected. Additionally, the quantity of generated ROS depended on the delivered dose. To prevent 1 O 2 およびO 2 。 − production possibly engendered by inadvertent exposure of water-soluble C60 (OH)46 to white light—which could have compromised the experiments in this study [60]—subsequent PDT experiments were conducted in the dark. This study focused on the quantities of generated 1 O 2 およびO 2 。 − 。 The water-soluble C60 (OH)46 exhibited considerable antibacterial effects, demonstrating its potential for application in PDT. Notably, after the same experiment, the water-soluble C60 (OH)21 (Additional file 1:Figs. S3, S4; Fig. 3a) was less effective in forming 1 O 2 およびO 2 。 − when compared with the water-soluble C60 (OH)46 (Additional file 1:Table S2). The water-soluble C60 (OH)46 generated more 1 O 2 およびO 2 。 − than did the water-soluble C60 (OH)21; additionally, the water-soluble C60 (OH)46 and water-soluble C60 (OH)21 had Φ Δ values of approximately 0.93 and 0.85, respectively (for reference, Φ Δ =0.64 is the QY of TSPP dissolved in D2 O [29, 30]).
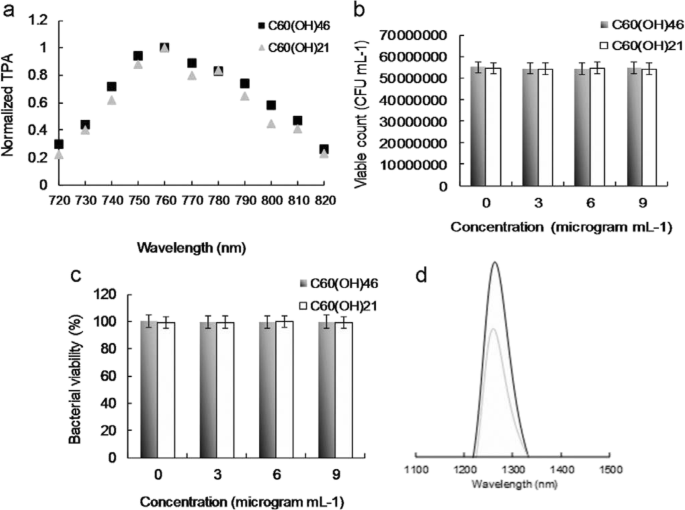
a Relative TPA spectra of the material. TPE as a function of the wavelength (720–820 nm) at 98.56 nJ pixel −1 that was used to monitor the signals. Delivered dose, 3 μg mL −1 water-soluble C60 (OH)46 またはC 60 (OH)21 fullerenol. The number of surviving b material-treated bacteria was determined by CFU counting assay and is expressed as the percentage (%) for c bacteria that corresponds to the unit of CFU mL −1 。 Delivered dose, OD600 ~ 0.05 of E.コリ and 0–9 μg mL −1 water-soluble fullerenol. d Measurement of phosphorescence spectra at 1270 nm for material. Delivered dose, 3 μg mL −1 water-soluble fullerenol. Data are means ± SD (n =6)
Antimicrobial Ability Determination Using TPE
Before the execution of antimicrobial experiments, the toxicity of water-soluble fullerenols must be examined to exclude factors that could contribute to bacterial elimination and confound experimental results. In addition, to prevent possible ROS production engendered by the inadvertent exposure of experimental materials to white light, which could confound experimental results [35], PDT experiments must be conducted in the dark. This study applied Gram-negative E.コリ as the experimental template. A CFU counting assay was conducted to determine the number of surviving bacteria (expressed herein as a percentage, corresponding to CFU mL −1 )。 The bacteria were treated with two types of the prepared water-soluble fullerenols (dose range, 0 to 9 μg mL −1 ) and incubated in the dark for 3 h at 37 °C to determine absorbance at 600 nm (OD600 ~ 0.05; Additional file 1:Fig. S1). The growth levels of the bacteria treated with the water-soluble fullerenols were first monitored by measuring absorbance at 600 nm. The initial absorbance was 0.05 OD600 , and the absorbance associated with both materials reached approximately 0.37 over time. Accordingly, neither material inhibited bacterial proferation. Moreover, the materials engendered a nearly 0 log10 reduction in the number of surviving bacteria (Fig. 3b), corresponding to a viability of approximately 100% (Fig. 3c). Accordingly, the materials were determined to exhibit excellent biocompatibility with the bacteria. Consequently, the materials subjected to 3 h of incubation in the dark at 37 °C were used to conduct experiments. Although the water-soluble fullerenol could generate ROS, interactions between materials and reagents (i.e., SOSG, t -MVP, XTT, and GSH) may result in false-positive ROS signals, thereby confounding PDT results [52]. Therefore, to exclude this possibility, bacteria were introduced and treated with materials in the present study. The amount of ROS generated from the photoexcited material-treated E.コリ 観察されました。 Table 2 presents the observed amount of ROS, revealing a similar trend to that in Tables S2–S3 (Additional file 1:materials alone and material-treated-Gram-positive Bacillus subtilis (B. subtilis )); these results were consistent with the 1 O 2 phosphorescence signal emitted from the materials at 1270 nm (Fig. 3d). PDT against E.コリ was performed using irradiation with a low dose of energy (211.2 nJ pixel −1 with 800 scans, total effective exposure time ~ 3.2621 s; Ex, 760 nm). The effects PDT on the viability of E.コリ treated with two-photon photoexcited materials were then determined (Fig. 4). No bactericidal effects were observed on bacteria alone (with or without laser exposure) or on the panel of material-treated bacteria without laser treatment (Fig. 4a). After TPE, bacterial viability was relatively low; specifically, the viability observed for the panel that was treated with the water-soluble C60 (OH)21 was nearly 15%, corresponding to an approximately 0.823 log10 reduction (Fig. 4b). By contrast, the bacterial viability observed for the panel treated with the water-soluble C60 (OH)46 was approximately 0 (100% elimination efficiency, corresponding to a ~ 7.736 log10 reduction). When the dose was increased, complete bactericidal effects were observed for both materials (Fig. 4c, d). However, antimicrobial effects did not differ by bacteria type (Gram-negative E. coli or Gram-positive B.枯草菌 ) after photoexcitation (Additional file 1:Fig. S5). In addition, regarding the fullerenols that eliminated bacteria, a higher composition of hydroxyl groups increased bactericidal capability when compared with a lower composition under identical treatment conditions.
<図> 図>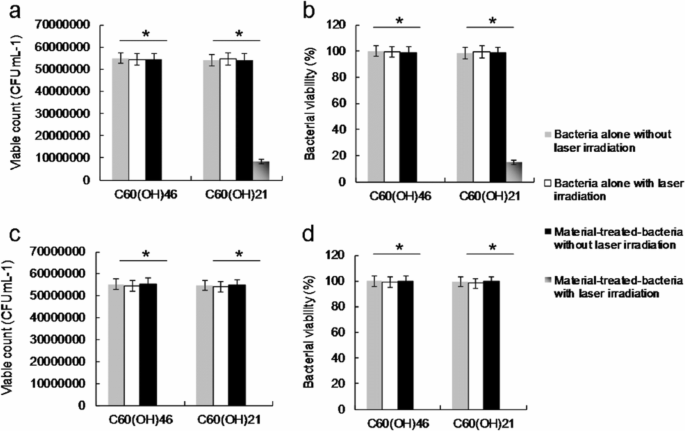
Viability (%) was quantified according to the determined viable count of material-treated bacteria through a CFU assay conducted using short excitation with a TPE power of 211.2 nJ pixel −1 with 800 scans (approximately 3.2621 s of total effective exposure time; Ex, 760 nm) to deliver a dose of a 、 b 3 or c 、 d 6 μg mL −1 。 Delivered dose, OD600 0.05 of E.コリ 。 Data are presented as means ± SD (n =6). For C60 (OH)46 - and C60 (OH)21 -treated E.コリ with photoexcitation, a p <0.001 and p =0.662, b p <0.001 and p =0.658, c p <0.001 and p <0.001, and d p <0.001 and p <0.001. * p value obtained by Student’s t テスト
Observation of Water-Soluble Fullerenol-Treated E.コリ Using TEM and Investigation of Two-Photon Properties
To observe the disruption of material-treated bacteria after photoexcitation, the water-soluble C60 (OH)46 with high PDT efficiency was selected, and bacteria were imaged using TEM. Bare E.コリ (Fig. 5a) were incubated with the water-soluble fullerenol for 3 h, resulting in the substantial adsorption of materials on the bacterial surfaces. Nevertheless, no unusual morphologies were observed, indicating normal live bacterial morphology (Fig. 5b). Uptake assay results revealed the adsorption of materials onto the bacterial surface, with the corresponding burst rate being approximately 85% within the first 3 h of incubation (Fig. 5c); the rate reached saturation from the 3rd to the 10th hour. Therefore, the materials were adsorbed and formed an external barrier on the bacterial surface. However, the E.コリ exhibited a distorted appearance and severe morphological changes over 3 days of incubation (Fig. 5d), resulting in a 0.940 log10 reduction that corresponded to a nearly 18% viability (Fig.5f; Additional file 1:Fig. S6). Material absorption and coating on the bacterial surface suppressed the absorption of nutrients essential for microbial growth and engendered changes in membrane (wall) permeability, thereby inducing internal osmotic imbalances and inhibiting microbial growth. In other words, the water-soluble fullerenol had antibacterial (bacteriostatic or bactericidal) effects after 3 days of incubation. Furthermore, the photoexcited material-treated bacteria, particularly E.コリ , exhibited unique morphologies with severe damage after 3 h of incubation (Fig. 5e, f; Additional file 1:Fig. S6). No heat-generated bubbles formed on the bacterial surface incurred damage, indicating that the water-soluble fullerenol did not have photothermal-mediated heat properties after photoexcitation (Additional file 1:Fig. S7). The viability of E.コリ was also determined through fluorescence and quantification (Fig. 6). The green fluorescence indicative of living bacteria in Fig. 6a reveals that the bacteria exposed to laser treatment alone were largely undamaged, which is consistent with the results presented in Fig. 5a. Dead bacteria were detectable after treatment with the materials and laser exposure (red fluorescence in Fig. 6b), a finding that is also consistent with that in Fig. 5e. Bacterial viability was quantified for further antimicrobial testing. Nearly complete elimination of the material-treated bacteria (Fig. 6c) was observed. Viability was also quantified using a CFU assay (Figs. 4a, b and 5f, and Additional file 1:Fig. S6) to demonstrate the antibacterial efficiency of the water-soluble C60 (OH)46 in PDT. According to the results in Figs. 4, 5, and 6; Table 2; and Table S2 (Additional file 1), E.コリ treated with the water-soluble C60 (OH)46 was susceptible to photoexcitation, leading to a higher death rate, increased ROS generation, and more severe morphological collapse compared with E.コリ treated with the water-soluble C60 (OH)21 。 In general, the absolute cross section for TPE makes fluorophores efficient for nonlinear microscopic studies because the ratio of the energy absorbed to the input energy flux to a specimen is high, thereby minimizing possible photodamage to specimens [39, 40]. When two-photon techniques are used to image molecular activities in living biological preparations and turbid tissues, a favorable cross section is desirable [61]. In the present study, the absolute cross section for TPE calculated for the water-soluble C60 (OH)46 was approximately 1037 GM (Goeppert-Mayer unit, with 1 GM =10 −50 cm 4 s photon −1 ) at a 760-nm excitation wavelength (fluorescein was the standard reference for the cross section [39, 40]; Fig. 1b and Tables 1 and 3); the absolute cross section calculated for the water-soluble C60 (OH)21 was approximately 1230 GM, which is similar to values obtained in relevant studies [62, 63]. These absolute cross sections could facilitate the two-photon process. Moreover, the fluorescence of the water-soluble C60 (OH)46 was illuminated through a two-photon process (Fig. 1b). The relative fluorescence QY was approximately 0.02 (the QY of Cy5.5 in dimethyl sulfoxide [31] served as a reference:QYref =0.28); similarly, the absolute QY [64] was approximately 0.01, and the same QYs were derived for one-photon excitation and TPE [31]. By contrast, the water-soluble C60 (OH)21 had lower relative and absolute QYs (0.06 and 0.05, respectively). In addition, this study investigated the lifetime of the fullerenols. The effects of radiative and nonradiative decay rates on QY and lifetime were calculated. The average lifetime of the water-soluble C60 (OH)46 was approximately 7.797 ns, as calculated from observed lifetimes of 0.149, 1.775, and 19.679 ns; the average lifetime of the water-soluble C60 (OH)21 was approximately 5.251 ns (Fig. 7 and Table 4). Therefore, the ratio of radiative to nonradiative decay rates of the water-soluble C60 (OH)46 was approximately0.020 (derived from rates of approximately 2.565 × 10 6 s -1 to 1.257 × 10 8 s -1 ), whereas that of the water-soluble C60 (OH)21 was approximately 0.064 (approximately 1.143 × 10 7 s -1 and 1.790 × 10 8 s -1 ; Additional file 1:Table S4). This finding is attributable to the existence of a hydroxyl group on the surface of the water-soluble fullerenol, which induced the nonradiative recombination of electron–hole pairs, leading to the inhibition of intrinsic state emission. However, hydroxylgroups at the edge of the water-soluble fullerenol may have a high occupied molecular orbital. This can be attributed to the strong orbital interaction between hydroxyl groups, which could thus increase the efficiency of intersystem crossing (rather than fluorescence generation) and generate numerous nanomaterial triplets with a high composition of hydroxyl groups; therefore, this would result in a high Φ Δ value for the water-soluble fullerenol and induce the fullerenol to react with oxygen according to the Jablonski diagram [65]. Consequently, two-photon PDT can be effectively performed using ultralow energy in an extremely short time, thereby providing an alternative approach to killing malignant species.
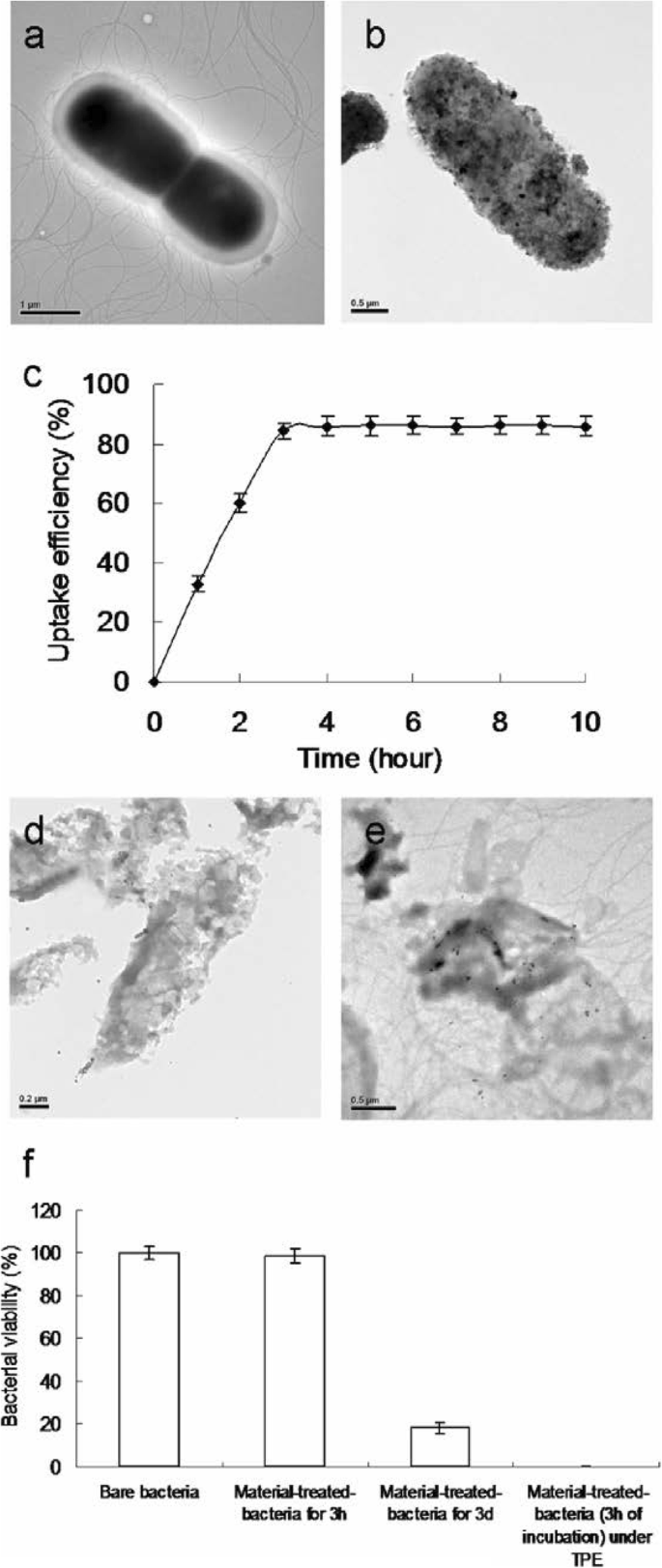
TEM images. a Showing bare bacteria without any treatment. Bacteria treated with material for b 3 h and d 3 days of incubation. e The photoexcited material-treated bacteria (3 h of incubation) with a TPE power of 211.2 nJ pixel −1 with 800 scans (approximately 3.2621 s of total effective exposure time; Ex, 760 nm). c Uptake assay of bacteria and material at 37 °C. f Viability (%) was quantified following the determined viable count of material-treated bacteria via CFU assay by short excitation with the same treatment. Delivered dose OD600 ~ 0.05 of E.コリ and 3 μg mL −1 water-soluble fullerenol C60 (OH)46 。 Data are means ± SD (n =6)
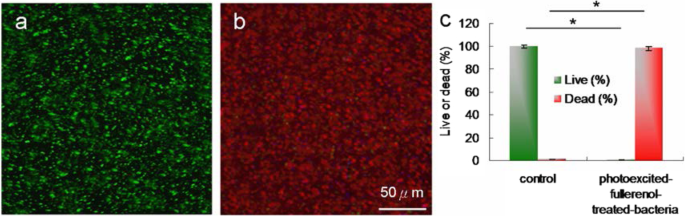
Images obtained after laser photoexcitation exposure (211.2 nJ pixel −1 ) with 800 scans (approximately 3.2621 s of total effective exposure time; Ex, 760 nm) of a 、 b material-treated bacteria. The Live/Dead kit was used to stain bacteria before images were obtained. Scale bar, 50 μm. c Viability (%) determination results. Delivered dose, OD600 ~ 0.05 of E.コリ and 3 μg mL −1 water-soluble fullerenol C60 (OH)46 。 For the percentages alive and dead, p <0.001. * p value obtained using Student’s t テスト。 Data are presented as mean ± SD (n =6)
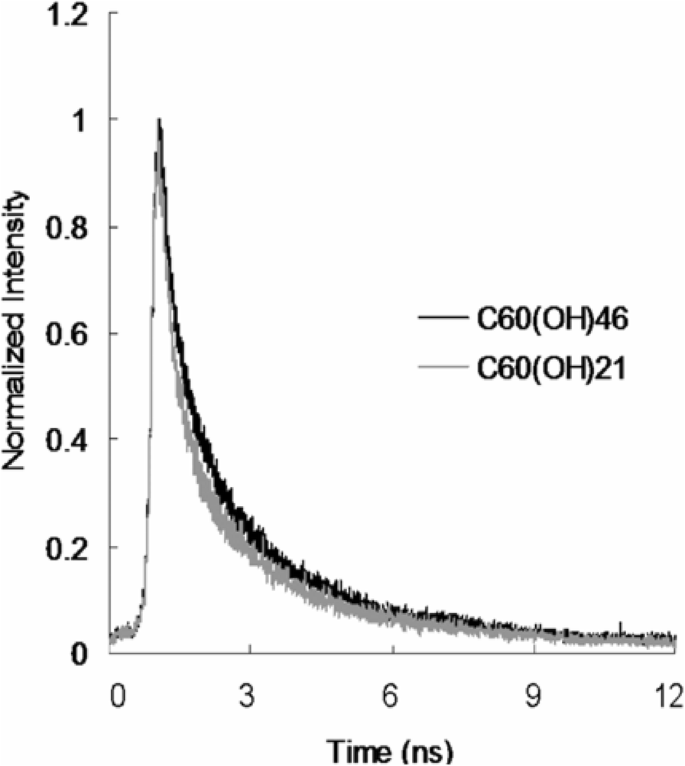
Time-resolved room-temperature PL decay profiles of material (98.56 nJ pixel −1 )。 Excitation wavelength, 760 nm. Delivered dose, OD600 ~ 0.05 of E.コリ and 3 μg mL −1 材料。 Data are presented as means ± SD (n =6)
結論
This study revealed that a water-soluble fullerenol material with a higher composition of hydroxyl groups had superior photoproperties to those of a fullerenol material with a lower composition of hydroxyl groups; the superior photoproperties can be attributed to the reduced laser exposure and materials used for treatment. Furthermore, the water-soluble fullerenol with a higher composition of hydroxyl groups exhibited high TPA, a favorable absolute cross section for TPE, and high two-photon stability. Therefore, this fullerenol has potential as a two-photon PS in two-photon PDT coupled with TPE. This property is probably due to the presence of a hydroxyl group on the surface of the water-soluble fullerenol, which caused the nonradiative recombination of electron–hole pairs, leading to the inhibition of intrinsic state emission. Moreover, hydroxyl groups at the edge of the water-soluble fullerenol may have a high occupied molecular orbital; this may be ascribed to the strong orbital interaction between the hydroxyl groups, thereby increasing intersystem crossing (rather than fluorescence generation) efficiency and generating numerous material triplets with a high composition of hydroxyl groups. Therefore, the water-soluble fullerenol would have a high Φ Δ value and react with oxygen according to the Jablonski diagram. Consequently, two-photon PDT can be effectively performed using ultralow energy in an extremely short time. Accordingly, this efficient alternative approach to managing malignant species presents possibilities for future clinical applications.
データと資料の可用性
All datasets are presented in the main paper.
略語
- PS:
-
光増感剤
- QY:
-
量子収率
- PDT:
-
光線力学療法
- UV–vis:
-
紫外可視
- ROS:
-
活性酸素種
- ψ Δ :
-
Singlet oxygen QY
- NIR:
-
近赤外線
- TPE:
-
Two-photon excitation
- E.コリ :
-
大腸菌
- HR-TEM:
-
高分解能透過型電子顕微鏡
- DLS:
-
動的光散乱
- FTIR:
-
フーリエ変換赤外
- XPS:
-
X線光電子分光法
- FD:
-
Field desorption
- CFU:
-
Colony forming unit
- TSPP:
-
Meso -tetra(4-sulfonatophenyl)porphine dihydrochloride
- PL:
-
フォトルミネッセンス
- DMSO:
-
ジメチルスルホキシド
- OPE:
-
One-photon excitation
- TPA:
-
Two-photon absorption
- TPL:
-
Two-photon luminescence
- ti-sa:
-
Titanium-sapphire
- NA :
-
Numerical aperture
- FLIM:
-
Fluorescence lifetime imaging microscopy
- 1 O 2 :
-
Singlet oxygen
- SOSG:
-
Singlet Oxygen Sensor Green
- t -MVP:
-
Trans-1-(2′-methoxyvinyl)pyrene
- O2 。 − :
-
Superoxide radical anion
- XTT:
-
2, 3-Bis (2-methoxy-4-nitro-5-sulfophenyl)-2H-tetrazolium-5-carboxanilide
- GSH:
-
Glutathione, γ -l-glutamyl-l-cysteinyl-glycine
- S1 :
-
Singlet
- T1 :
-
Triplet
- B。枯草菌 :
-
枯草菌
- GM:
-
Goeppert-Mayer
ナノマテリアル
- 電気触媒水素発生のための制御された厚さのMoS2
- 効率的な光触媒水素生成のためのS、N共ドープグラフェン量子ドット/ TiO2複合材料
- 化学センシング用の貴金属ナノ粒子で装飾されたエレクトロスピニングポリマーナノファイバー
- スーパーキャパシターの電極材料としての階層的多孔質構造を持つ単分散カーボンナノスフェア
- 水素製造用の効率的な光触媒としてのZr-金属-有機フレームワークの探索
- 非常に効率的な光触媒水素発生のためのZnO @ TiO2中空球の階層的ヘテロ構造
- 最大の太陽エネルギー収穫のためのInPナノワイヤの効率的かつ効果的な設計
- SドープSb2O3ナノ結晶:有機分解用の効率的な可視光触媒
- 逆ピラミッド微細構造を備えた20.19%効率の単結晶シリコン太陽電池の製造
- FoxChaseはRodonGroupと提携して、テンプルヘルス用のCOVIDテストスワブを開発しています
- ボルボグループは、自律型トラック用のAIプラットフォームでNvidiaと提携しています