F-アクチンは、MKL1およびYAP / TAZを介してTiO2ナノチューブ上の間葉系幹細胞の骨芽細胞分化を調節します
要約
チタンおよびチタン合金は、整形外科用インプラントで広く使用されています。ナノトポグラフィーを変更すると、チタン基板のオッセオインテグレーションを改善するための新しい戦略が提供されます。フィラメント状アクチン(F-アクチン)重合は、機械的負荷構造として、一般に、細胞移動、エンドサイトーシス、細胞分裂、および細胞形状の維持に関与していると考えられています。 F-アクチンが関与しているかどうか、およびそれが間葉系幹細胞(MSC)のナノチューブ誘発性骨形成分化にどのように機能するかはまだ解明されていません。この研究では、TiO 2 を製造しました 陽極酸化によるチタン基板の表面上のナノチューブは、走査型電子顕微鏡(SEM)、X線エネルギー分散型分析(EDS)、および原子間力顕微鏡(AFM)によってその特徴を特徴づけます。アルカリホスファターゼ(ALP)染色、ウエスタンブロッティング、qRT-PCR、および免疫蛍光染色を実施して、骨形成能、F-アクチンのレベル、およびMKL1とYAP / TAZの発現を調べました。結果は、TiO 2 の内径と粗さを示しました。 ナノチューブは、陽極酸化電圧が30Vから70Vに増加するにつれて増加しましたが、その高さは一貫して2μmでした。さらに、チューブの直径が大きいほど、TiO 2 の能力が強くなります。 MSCの骨形成分化を促進するナノチューブ。 Cyto DによるF-アクチン重合の阻害は、MSCの骨形成分化、ならびにビンキュリン(VCL)や接着斑キナーゼ(FAK)などの接着斑複合体に含まれるタンパク質の発現を抑制しました。対照的に、Jasp処理後、F-アクチンの重合はRhoAと転写因子YAP / TAZの発現を増強しました。これらのデータに基づいて、TiO 2 ナノチューブはMSCの骨形成分化を促進し、この能力は、特定の範囲(30〜70 V)内でナノチューブの直径が大きくなるにつれて強化されました。 F-アクチンは、MKL1とYAP / TAZを介してこのプロセスを仲介しました。
はじめに
チタンおよびチタン合金は、その優れた生体適合性、耐食性、および機械的特性により、人工関節全置換術や歯科インプラントなどの臨床用途で広く使用されています[1,2,3]。しかし、無菌性の緩みや感染など、まだ解決すべき課題がたくさんあります[4、5]。近年、オッセオインテグレーションと抗菌性を高めることを目的とした多くの研究が行われています。たとえば、MoS 2 チタンインプラントの/ PDA-RGDコーティングは、チタンインプラントと宿主の骨との統合を促進するだけでなく、細菌の増殖を高効率で阻害することができます[6]。さらに、表面トポグラフィーはますます注目を集めており、トポグラフィーの変更は、マイクロおよびナノスケールの構造を変更するだけで化学的な変更とは異なります。細胞への化学信号の刺激は不安定で細胞毒性があります。それに比べて、安全で制御可能な物理信号は、化学分子によって引き起こされるいくつかの副作用を回避することができます。したがって、インプラントの表面の地形的改変および地形的構造による骨統合の調節は、プロテーゼの移植後の不十分な骨統合の臨床的問題を解決するための新しい方法を提供することができます。
骨組織工学と骨再生の分野では、細胞と形態の相互作用は、種子細胞の機能と分化を正確に制御するための有望な管理戦略であると考えられています。同時に、骨自体はナノメートルとミクロンの範囲内でエレガントな階層を持っています[7]。したがって、表面形態は、自然の骨構造を模倣し、宿主の骨およびインプラントの表面にある間葉系幹細胞の骨形成分化を促進することができる同様のニッチを提供することができます。表面形態は、ナノチューブ、ナノワイヤー、ナノポアなどを含む多くの異なる構造で構成することができます。特に、ナノチューブアレイは、高い表面積対体積比、生物学的可塑性、高い吸着容量などの独自の表面特性により、近年多くの分野で大きな関心を集めています。たとえば、新しい研究では、窒化ホウ素ナノチューブ(BNNT)がガスに敏感な材料を構成し、オイル中の溶存ガスの組成と含有量を検出することで変圧器の動作を監視するガスセンサーとして使用できることが示されています[8]。生物医学では、表面トポグラフィーは、細胞の移動、接着、増殖、分化などの細胞の挙動を指示することもできます。最新の研究では、ナノスケールのトポグラフィーが間葉系幹細胞(MSC)を骨芽細胞に分化させ、初期のオッセオインテグレーションを強化できることが明らかになっています[9、10、11、12]。マイクロスケールとナノスケールの表面修飾を組み合わせることで、MSCが収縮性平滑筋細胞に分化する可能性があることも報告されています[13]。ただし、表面トポグラフィーが細胞の運命をどのように導くかについての分子メカニズムはまだ解明されていません。これは、材料の安全性評価と材料設計にとって重要です。
フィラメント状(F)-アクチンは、マイクロフィラメントとも呼ばれ、真核細胞の細胞骨格の3つの主要な構成要素の1つです。これは、球状(G)-アクチンのポリマーで構成されており、他の多くのタンパク質によって修飾されています。 F-アクチンは、すべてのマイクロフィラメントのサブユニットが同じ端を向いているため、構造的な極性があります。とげのある端は別の隣接するモノマーに向けられ、尖った端はATP結合部位が露出したアクチンサブユニットを持っています。つまり、ATPはG-アクチンとF-アクチンの間の変換プロセスに関与しています。このプロセスは動的平衡状態にあり、重合と解重合が同時に起こります。これはトレッドミリングとも呼ばれ、葉状仮足と糸状仮足でよく見られます[14]。したがって、アクチンダイナミクスは、細胞移動、細胞分裂、細胞形状の維持などの細胞機能において重要な役割を果たしていることは明らかです。ただし、F-アクチンは、機械的負荷をサポートする物理的構造として機能するだけでなく、シグナル伝達や遺伝子発現などの他の生物学的挙動にも関与します。蓄積された証拠は、F-アクチンが他のタンパク質と相互作用することによって物理的信号を化学的信号に変換できることを示しています[15、16、17、18]。たとえば、生体力学的および幾何学的な再構築は、アクチンモノマーの重合がF-アクチンに重なるのを防ぐことにより、腫瘍細胞のアポトーシスを促進します[15]。超音波パルスは、F-アクチンの解重合を阻害することにより、ヒト間葉系幹細胞の骨形成を促進します[16]。私たちの以前の研究はまた、機械的ひずみがF-アクチンの安定性を高めることを示しました[17]。ナノチューブの中空構造は細胞の接着部位が少ないため、生体力学的バランスを維持するために細胞骨格の再配列は避けられません。したがって、F-アクチンがナノトポグラフィーによって誘導される細胞分化を媒介する可能性が高いと確信する理由は間違いありません。
この研究では、TiO 2 を製造しました ナノチューブは、陽極酸化によってトポグラフィーを変更し、MSCの骨形成分化を促進する能力を調査しました。次に、F-アクチンがメカノトランスダクションに重要な役割を果たすかどうかを調べました。サイトカラシンD(Cyto D)は、F-アクチンのとげのある末端に競合的に結合して、G-アクチンがフィラメントに組み込まれるのを防ぎ、F-アクチンの重合を阻害するために使用され、ジャスプラキノリド(Jasp)は、アクチンアセンブリ。さらに、物理的な手がかりを生化学的信号に変える際にF-アクチンがどのように機能するかも解明したかった。以前の研究の結果に基づいて、MAPK経路がこのプロセスに関与している可能性があると仮定しました[17]。はい関連タンパク質(YAP)/ PDZ結合モチーフ(TAZ)を備えた転写コアクチベーターやMKL1などの転写因子も、F-アクチンが幹細胞にどのように影響するかをスクリーニングするための研究の対象でした。他の分野でのいくつかの研究は、それらがF-アクチンに関連していることを示唆しているため、運命です[19、20、21]。一般的に、ナノチューブによって修飾されたインプラントの材料設計とバイオセーフティ評価を導くために、ナノチューブによって誘発される幹細胞分化の過程におけるF-アクチンの役割を明らかにしたいと考えています。
材料と方法
TiO 2 の製造 ナノチューブ
基板として使用された純チタンスライス(純度99.9%、厚さ2 mm、Shengshida、河北省、中国)は、400番と1500グリットの炭化ケイ素サンドペーパーで研磨されました。次に、サンプルを超音波洗浄機でアセトン、無水アルコール、脱イオン水で順次洗浄し、最後に室温で3時間乾燥させました。ナノトポグラフィーを作製するために、前処理したサンプルをアノードとして固定し、0.15 M NH 4 の電解質水溶液でカウンターカソードとして白金片を使用しました。 Fおよび90%グリコールを1時間。陽極酸化電圧は30、40、50、60、または70 Vの定電圧でした。陽極酸化後、すべてのサンプルを脱イオン水で30分間すすぎ、超音波洗浄機で15分間無水アルコールで洗浄しました。最後に、すべてのサンプルをオートクレーブで120°Cで1時間滅菌し、使用前に培地で湿らせました。
ナノチューブ製造の反応メカニズムは明確ではなく、現在の主流の理論はフィールド強化溶解理論です。ナノチューブアレイの形成は、場の酸化、場の溶解、および化学的溶解の作用下での動的平衡の結果です(図1b)。陽極酸化プロセスは次のように説明できます。最初のステップでは、電解質と金属の界面に酸化物バリア層が形成されます。
$$ {\ mathrm {Ti}} ^ {4 +} + 2 {\ mathrm {H}} _ 2 \ mathrm {O} \ to {\ mathrm {Ti} \ mathrm {O}} _ 2 + 4 {\ mathrm { H}} ^ {+} $$(1)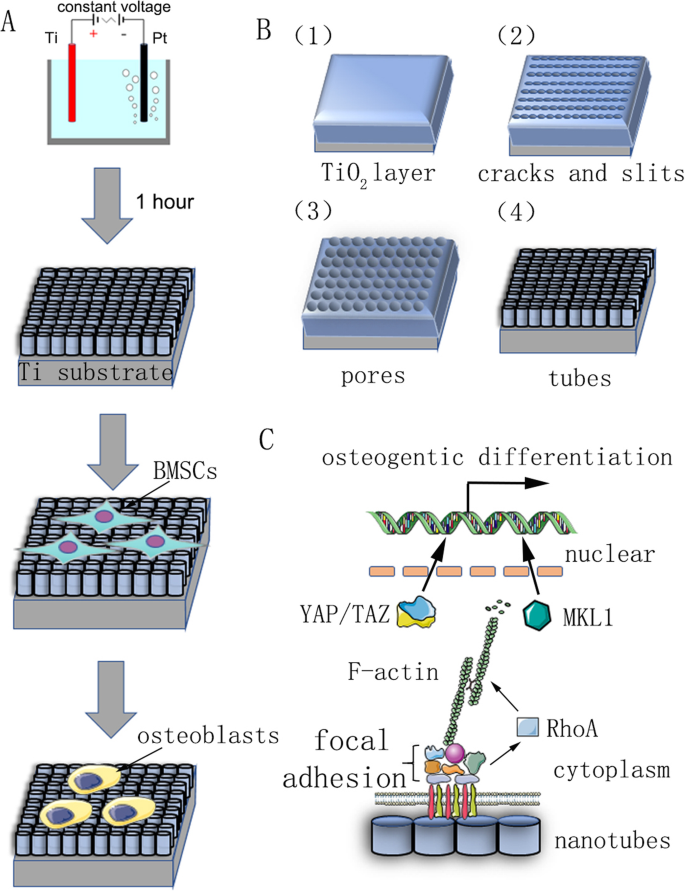
私たちの研究の要約チャート。 a 陽極酸化と細胞分化の誘導を示すフローチャート。 b 前処理されたチタン基板は、0.15 M NH 4 の電解質水溶液のアノードとして固定されました。 Fおよび90%グリコール、定電圧で1時間。自己組織化ナノチューブは均一に形成されると予測された。その反応メカニズムは、材料と方法で説明されています。 c ナノチューブによって誘導される幹細胞の骨形成分化のメカニズムの概略図
次に、酸化物層の電界増強溶解により、表面に亀裂と狭いスリットが現れます。 F − の拡散 これらの亀裂やスリットへのイオンは、溶解速度を高めます。亀裂は拡大し、隣接する亀裂とつながります。最後に、酸化チタン層の形成速度と溶解速度が動的バランスに達し、ナノチューブは成長しなくなります。
$$ {\ mathrm {TiO}} _ 2 + 6 {\ mathrm {F}} ^ {-} + 4 {\ mathrm {H}} ^ {+} \ to {\ left [{\ mathrm {TiF}} _ 6 \ right]} ^ {2-} + 2 {\ mathrm {H}} _ 2 \ mathrm {O} $$(2)表面の特性評価
異なる電圧(30、40、50、60、または70 V)で製造されたサンプルは、エタノールと脱イオン水で15分間リンスされ、室温で乾燥されました。走査型電子顕微鏡(SEM450、FEI Nova Nano SEM、Thermo Fisher Scientific、マサチューセッツ州ウォルサム、米国)を使用して、表面構造を特徴付け、サンプルを金の薄層でコーティングした後のナノチューブの内径と高さを測定しました。一方、ナノチューブの元素組成を分析するために、X線エネルギー分散型分析(EDS)が実行されました。原子間力顕微鏡(AFM、NanoManVS、Bruker Nano Surfaces、Bruker MicroCT、Kontich、ベルギー)を使用して、サンプルの表面形態と表面粗さを調査しました。各サンプルから3つの異なる領域が選択され、測定が3回繰り返されました。
細胞培養
4週齢のオスのSprague-Dawley(SD)ラットは、上海第9人民病院(中国、上海)の実験動物センターから購入しました。ラット骨髄間葉系幹細胞(BMSC)は、大腿骨と脛骨から無菌的に分離されました。 BMSCを精製し、10%(v / v)ウシ胎児血清(FBS)を含むα-最小必須培地(α-MEM; Hyclone、Logan、UT、USA)(Gibco / Life Technologies、Carlsbad、CA、USA)でさらに増殖させました。 )、100 mg / mLストレプトマイシン(Gibco)、および100 U / mLペニシリン(Gibco)、95%空気および5%CO 2 からなる加湿雰囲気で37°Cで培養 。培地は2日ごとに更新され、細胞はトリプシン処理され、80%のコンフルエンスで継代培養されました。この研究で使用したすべての細胞は、継代3〜5でした。骨形成誘導培地は、100 nMデキサメタゾン、10mMβ-グリセロホスフェート、および50 mMアスコルビン酸を添加した増殖培地で構成されました(Sigma-Aldrich、セントルイス、ミズーリ州、米国)。 。
細胞増殖アッセイ
TiO 2 ナノチューブで修飾されたチタンスライスを円形に切断し、24ウェル細胞培養プレートのウェルに配置しました。 3〜5継代のBMSCは、TiO 2 で培養されました。 3×10 4 の密度のナノチューブ 増殖培地または骨形成培地のいずれかにおける細胞/ディスク。細胞培養の2日後、F-アクチン重合を妨害するために使用されるサイトカラシンD(Cyto D、Sigma-Aldrich)およびジャスプラキノリド(Jasp、Sigma-Aldrich)を3日間毎日培地に添加しました。 Cyto DとJaspの最終濃度と作業時間はそれぞれ5μM、1時間と2μM、3時間でした。試薬とのインキュベーション後、培地を更新した。 Cyto DまたはJasp処理の12時間後に、Cell Counting Kit-8(CCK8)アッセイ(Dojindo、熊本、日本)を使用して細胞の生存率と増殖を評価しました。細胞を10%(v / v)CCK8溶液とともに、セルインキュベーター内で37°C、5%CO 2 下で2時間インキュベートしました。 。次に、100μLの反応混合物を96ウェルプレートのウェルに移し、マルチスキャンUV-可視分光光度計(Safire2; TECAN、Mannedorf、Switzerland)を使用して培養中のホルマザン色素生成物の吸光度(OD)を450nmで測定しました。 )。さらに、自動セルカウンター(AMQAX1000、Life Technologies)を使用しておおよその細胞数もカウントしました。細胞を数える前に、BMSCはTiO 2 から酵素的に分離されました ナノチューブとトリパンブルー(Sigma–Aldrich)で染色。
アルカリホスファターゼ染色およびALP活性分析
BMSCは5つの異なるTiO 2 にシードされました 3×10 4 の密度のナノチューブ修飾チタンスライス(30、40、50、60、または70 V) ウェルあたり、骨形成培地で培養。上記のように試薬を加えた。 7日間のインキュベーション後、BMSCをTiO 2 で培養しました。 ナノチューブをPBSで3回洗浄し、4%パラホルムアルデヒドで固定し、ALPキットのアルカリホスファターゼ(ALP)作業溶液で、製造元の指示(Hongqiao、上海、中国)に従ってインキュベートしました。 PBSで洗浄した後、実体顕微鏡で結果を観察しました。
ALP活性分析では、まず細胞をプロテアーゼおよびホスファターゼ阻害剤を含まないRIPAバッファーで溶解し、次に遠心分離した溶解物をALPアッセイキット(Beyotime Institute of Biotechnology、江蘇省、中国)を使用して提供されたプロトコルに従ってアッセイしました。活性は最終的に対応するライセートのタンパク質濃度に正規化されました。
免疫細胞化学
Cyto DおよびJasp処理の3日後、BMSCを4%パラホルムアルデヒドで室温で20分間固定し、PBSで3回洗浄しました。細胞を0.3%Triton-X 100で30分間透過処理し、PBSで3回洗浄し、ローダミン結合ファロイジンで室温、暗所で1時間染色しました。次に、細胞をPBSでリンスし、DAPI(Beyotime Institute of Biotechnology)で室温で10分間対比染色しました。 PBSでさらに3回洗浄した後、サンプルをスライドガラスに固定し、共焦点顕微鏡で観察しました。
ウエスタンブロッティング
タンパク質発現を評価するために、TiO 2 で培養されたBMSC ナノチューブはトリプシン(Gibco)で収穫されました。細胞をPBSで3回洗浄し、プロテアーゼとホスファターゼ阻害剤カクテルを添加したRIPAバッファーで氷上で30分間溶解しました。 12,000× g での遠心分離により溶解物を回収した。 4°Cで15分間。上清中の総タンパク質濃度は、ビシンコニン酸(BCA)タンパク質アッセイキット(Beyotime)を製造元の指示に従って使用して測定しました。上記のようにローディングバッファーをタンパク質サンプルに加え、95°Cで15分間煮沸しました。ウエスタンブロッティング分析では、10μLのタンパク質調製物を12.5%SDS-PAGEゲル(EpiZyme Inc.、Cambridge、MA、USA)にロードし、120 Vで1時間電気泳動を行った後、ポリフッ化ビニリデンに電気転写しました。 (PVDF)膜を250mAで2時間。次に、メンブレンをTBST中の5〜10%脱脂粉乳で、シェーカー上で室温で1時間ブロックし、希釈バッファー(Beyotime)で希釈した一次抗体と4°Cで一晩インキュベートしました。次に、TBSTで5分間3回洗浄した後、希釈バッファーで希釈した蛍光標識二次抗体をメンブレンに添加し、メンブレンを室温で1時間暗所でインキュベートしました。タンパク質バンドは、2色の赤外線蛍光イメージングシステム(Odyssey、LiCor Biosciences、リンカーン、ネブラスカ、米国)によって検出されました。特に、内部参照タンパク質のバンドが統一されている場合は、メンブレンを剥がし、別の一次抗体で再プローブした後、同じプロセスを実行します。タンパク質発現を正規化するための内部参照タンパク質としてGAPDH抗体を使用し、この研究で使用した他の一次抗体は、抗ビンキュリン(1:1000希釈、Abcam、ケンブリッジ、マサチューセッツ州、米国)、抗FAK(1:1000希釈)でした。 、Cell Signaling Technology、Danvers、MA、USA)、anti-Runx2(1:1000希釈、Cell Signaling Technology)、anti-RhoA(1:1000希釈、Cell Signaling Technology)、anti-F-actin(1:500希釈) 、Abcam)、anti-Osx(1:500希釈、Abcam)、およびanti-pYAP(1:1000希釈、Cell Signaling Technology)。二次抗体は、ヤギ抗マウスIgG H&L(IRDye®680RD、1:5000希釈、Abcam)およびヤギ抗ウサギIgG H&L(IRDye®680RD、1:5000希釈、Abcam)でした。
定量的リアルタイムPCR
定量的リアルタイムPCRを7日目に実施し、ラント関連転写因子2(Runx2)、Osterix(Osx)、Alp、オステオカルシン(OCN)、RhoA、YAP、TAZ、ビンキュリン(VCL)、 TiO 2 上のオステオカルシンで増殖した細胞における限局性接着キナーゼ(FAK)、および巨核芽球性白血病1(MKL1) ナノチューブ。 Total RNA Kit(R6812-01HP、Omega Bio-Tek Inc.、Norcross、GA、USA)を使用して、細胞からトータルRNAを抽出しました。 RNAサンプルの濃度と純度は、波長260での光学密度によって決定され、A260 / 280比と1.8より高いA260 / 230比の両方を示すサンプルのみが分析されました。 qScript cDNA合成キット(宝、滋賀、日本)を製造元の指示に従って使用して、RNAサンプルをcDNAに逆転写しました。定量的リアルタイムPCRは、QuantStudio 6 FlexリアルタイムPCRシステム(Life Technologies)を使用してSYBR®PremixExTaq™(Takara)で実行されました。ハウスキーピング遺伝子であるGAPDHを内部参照として使用しました。データは、比較Ct(2 -ΔΔCt )を使用して分析されました。 )メソッドであり、コントロールと比較した倍率変化として表されます。使用したプライマーの配列を表1に示します。
<図> 図>統計分析
すべてのデータは、特に明記されていない限り、3つのサンプルを使用した少なくとも3つの独立した実験を表しています。データは平均±標準偏差(SD)として表されます。グループ間の差異は、一元配置分散分析とそれに続くStudent-Newman-Keuls事後検定またはStudent’s t によって評価されました。 テスト。 P <0.05の値は統計的に有意であると見なされました。
結果
表面の特性評価
ナノトポグラフィーを作成するには、TiO 2 ナノチューブは、異なる定電圧(30、40、50、60、および70 V)で1時間、陽極酸化装置を使用して純チタン基板上に形成されました(図1)。自己組織化ナノチューブの均一に分布したアレイが走査型電子顕微鏡(SEM)によって観察されました。ナノチューブの側面図と上面図を図2a、bに示します。この研究のすべてのサンプルのナノチューブの高さは約2μmでしたが、ナノチューブの内径は約74 nm(30 V)、92 nm(40 V)、112 nm(50 V)、128 nm(60 V)、および148 nm(70 V)(図2c)。これは、ナノチューブの高さが陽極酸化の時間に関連し、内径が陽極酸化の電圧に関連していることを示した。次に、X線エネルギー分散型分析(EDS)を実行して、ナノチューブの元素組成を分析しました。これは、ナノチューブがOとTiの2つの元素のみで構成されていることを示しています(図2d、e)。原子間力顕微鏡(AFM)を使用して、ナノチューブ構造を検出し、表面粗さ(Ra)としてナノチューブのプロファイルの算術平均偏差を測定しました(図2f)。データは、ナノチューブの表面粗さが直径(すなわち、陽極酸化の電圧)が増加するにつれて増加することを示しました(図2g)。
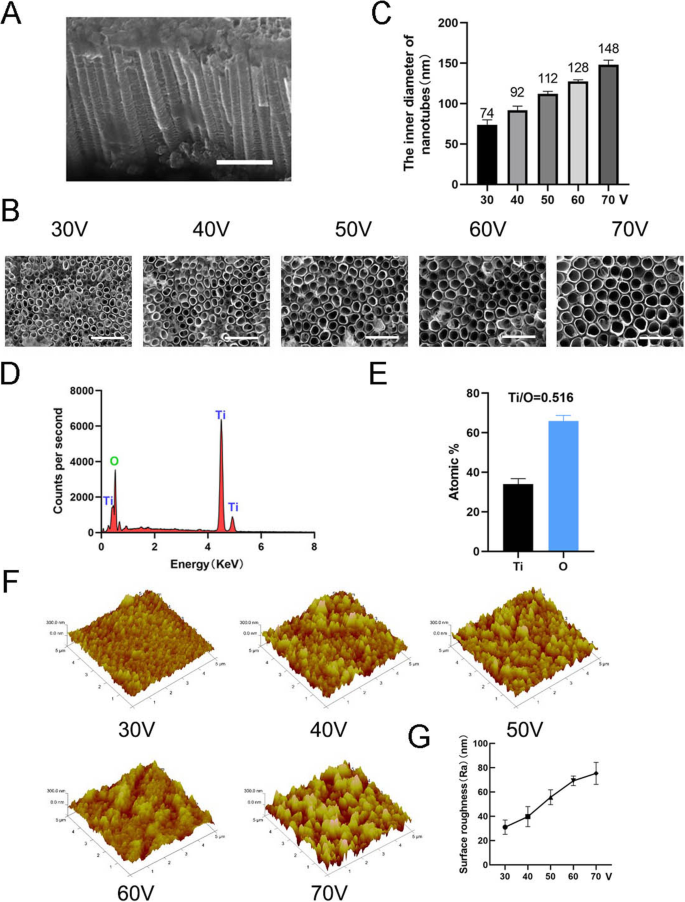
ナノチューブの表面特性。 a ナノチューブの側面図。スケールバー:1μm。 b ナノチューブの上面図。スケールバー:500nm。 c 5つの異なる定電圧(30、40、50、60、70 V)でのナノチューブの内径。 d ナノチューブ構造のEDS化学元素組成。 e TiとOの元素比。 f ナノチューブ構造の原子間力顕微鏡(AFM)画像。 g ナノチューブの平均表面粗さ(Ra)
TiO 2 ナノチューブはMSCの骨形成分化を誘導しました
骨誘導の7日後、MSCの骨形成分化を評価するためにALP染色を最初に実施した。染色結果は、MSCがTiO 2 で培養されたことを示しました。 ナノチューブは、滑らかなチタン基板上で培養された細胞(コントロールグループ)よりも高いALP活性を示しました(図3a)。染色領域の統計分析は、ナノチューブが骨形成分化を誘導する能力が、対照群と比較した場合に有意に増強されたことを示した。一方、この実験の直径範囲内では、TiO 2 の直径が大きくなる傾向が見られました。 ナノチューブは、骨形成分化を誘導する能力が強い(図3b)。したがって、70 Vグループは、結果をより適切に表示するために後続の実験で使用されました。次に、3日目と7日目の骨形成遺伝子発現を分析しました。TiO 2 で培養したMSC ナノチューブは3日間と7日間の両方で、対照群と比較して骨形成遺伝子(RUNX2、ALP、OCN、およびOSX)の発現の有意な促進を示しました(図3d–g)。ウエスタンブロットの結果により、RUNX2およびOSXのタンパク質発現も7日間の骨誘導後に増加したことが確認されました(図3c)。興味深いことに、F-アクチンがTiO 2 でアップレギュレーションされていることがわかりました。 ナノチューブグループ。したがって、TiO 2 ナノチューブは、MSCを骨芽細胞の分化に向けました。これはナノチューブの直径に関連していました。私たちの結果は、このプロセスにF-アクチンが関与していることも示唆しています。
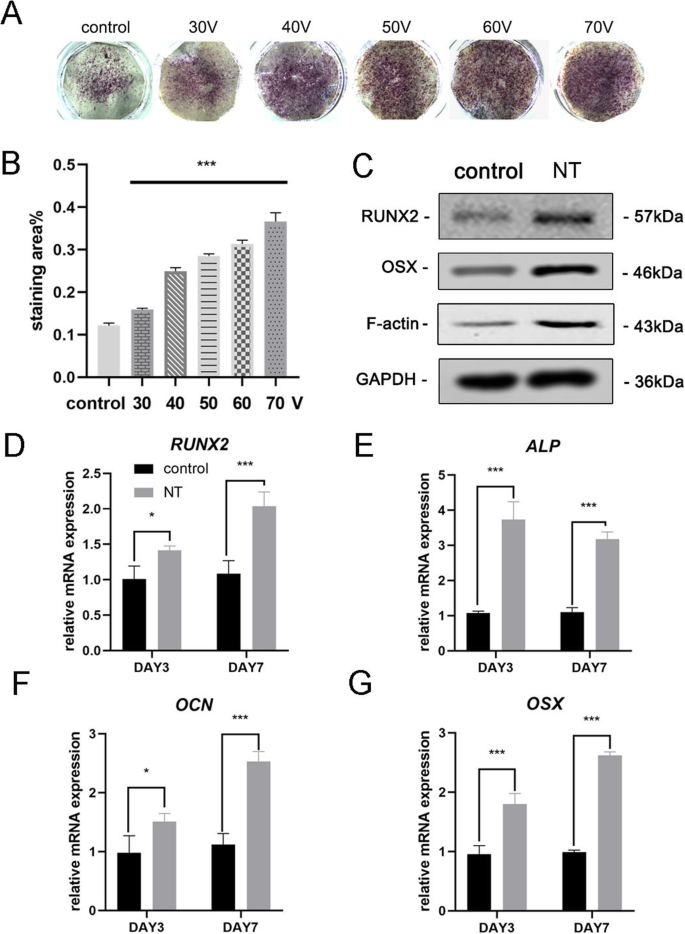
TiO 2 ナノチューブはBMSCの骨形成遺伝子発現を増強しました。 a 滑らかなチタン基板と5つの異なるナノチューブ基板のALP染色。細胞を骨形成培地で7日間誘導した。 b 染色領域の統計分析は、ImageJを使用して実行されました。 c MSCの骨形成関連タンパク質(RUNX2、OSX)とF-アクチンを7日目にウエスタンブロッティングで分析しました。RUNX2のmRNA発現( d )、ALP( e )、OCN( f )、およびOSX( g )3日目と7日目に、qRT-PCRによって分析されます。 NT ナノチューブグループ。データは、3つのサンプルの平均±SDを表します。 * P <0.05、** P <0.01、および*** P <0.001
F-アクチンを介したTiO上のMSCの骨芽細胞分化 2 ナノチューブ
F-アクチンがナノトポグラフィーによって誘導される細胞分化に関与しているかどうかをさらに調べるために、2つの試薬、ジャスプラキノリド(Jasp)とサイトカラシンD(Cyto D)を使用して、F-アクチンの重合をそれぞれ正と負の方法で調節しました。ローダミン-ファロイジン染色の共焦点顕微鏡写真は、Cyto D治療群のF-アクチンがほとんど解重合され、繊維状構造がほとんど見られないことを示しましたが、JaspはF-アクチンを安定化および重合し、観察されたよりも明確で明るい束のような構造によって確認されました対照群では(図5a)。さらに、ウエスタンブロット分析により、F-アクチンのタンパク質発現が影響を受けていることも確認され、Cyto DとJaspの両方が期待どおりに機能したことが証明されました(図5b)。細胞増殖アッセイは、Cyto Dが細胞増殖を有意に阻害し、Jaspが細胞増殖を促進することを示しました(図4a)。細胞数の結果はこの発見と一致していました(図4b)。
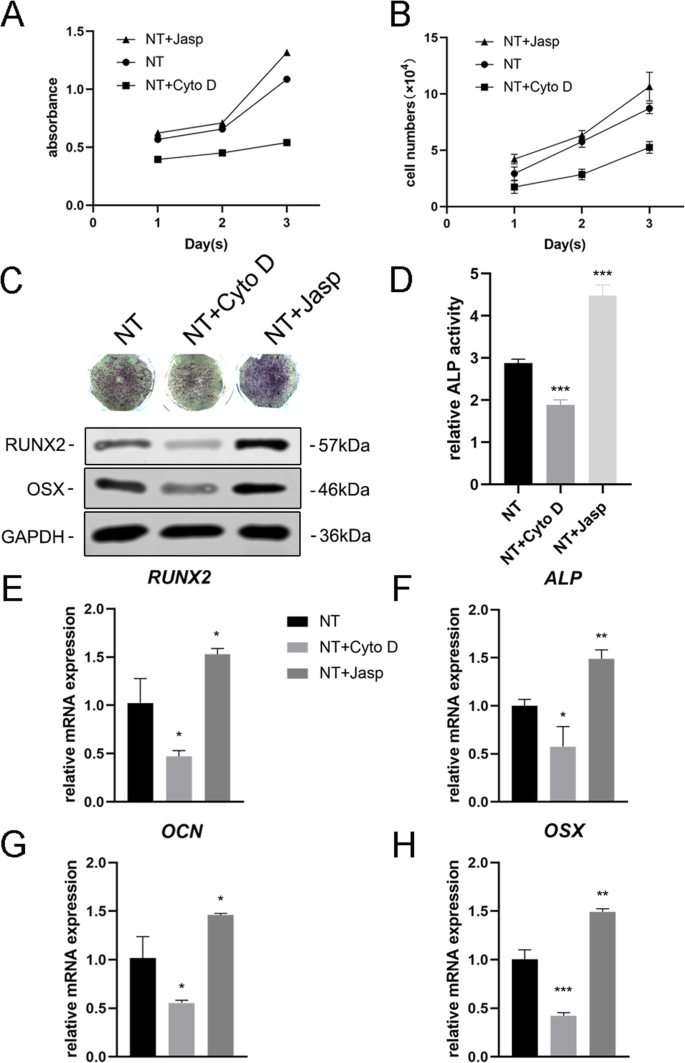
F-アクチンアセンブリは、BMSCの骨形成遺伝子の発現を調節しました。 a 、 b Cyto DおよびJasp処理後の細胞増殖は、1、2、および3日目にCCK-8アッセイまたは自動セルカウンターを使用して決定されました。 c 、 d ALP染色およびALP活性分析を実施して、3日間の薬物治療後のMSCにおけるALPの発現を評価しました。染色領域はImageJを使用して分析されました。 c 、 e – h ウエスタンブロッティングとqRT-PCRを使用して、NT + CytoDグループとNT + Jaspグループの骨形成関連マーカーの変化を対照グループ(薬物治療なし)の変化と比較しました。 NT ナノチューブグループ。データは、3つのサンプルの平均±SDを表します。 * P <0.05、** P <0.01、および*** P <0.001
次に、MSCが骨芽細胞に分化する能力を評価して、F-アクチンがこのプロセスを媒介したかどうかを調べました。我々は最初にALPを骨形成の初期マーカーとして検出しました。対照群と比較して、Cyto D治療はALPの発現とその活性を低下させましたが、Jasp治療群のそれはアップレギュレーションされました(図4c、d)。この結果と一致して、Jasp処理はRUNX2とOSXのタンパク質レベルの増加をもたらしましたが、Cyto Dは反対の効果をもたらしました(図4c)。これと一致して、RUNX2、ALP、OCN、OSXなどの骨特異的遺伝子のmRNA発現レベルは、薬物治療後に同じ傾向を示しました(図4e–h)。とりわけ、これらのデータは、F-アクチンがTiO 2 によって誘導されるMSCの骨形成分化の過程で重要な役割を果たしたことを示しています。 ナノチューブ。 F-アクチンの解重合を促進すると、ナノトポグラフィーによって誘発される骨芽細胞の分化が抑制され、F-アクチンの安定化と重合によって骨芽細胞の分化が促進されました。
F-アクチンによるTiO上のMSCの骨芽細胞分化 2 MKL1およびYAP / TAZを介したナノチューブ
MSCの運命の調節におけるF-アクチンが関与する根本的なメカニズムを分析するために、F-アクチンと直接相互作用するか、F-アクチンの重合に影響を与えるタンパク質/分子を調査しました。まず、ナノトポグラフィーがF-アクチンとG-アクチンのバランスにどのように影響するかを特定しようとしました。 TiO 2 物理的信号としてのナノチューブは、膜透過性の化学的信号とは異なるため、細胞膜のいくつかの成分を使用して刺激を細胞に伝達する必要があります。蓄積された証拠は、インテグリン、タリン、接着斑キナーゼ(FAK)、ビンキュリン(VCL)、テンシン、およびその他のタンパク質を含む接着斑複合体が、細胞外マトリックス(ECM)の状態について細胞に通知するシグナルキャリアとして機能することを示しています)したがって、それらの生物学的挙動に影響を及ぼします[22、23]。さらに重要なことに、F-アクチンはそのような接着斑複合体を介してインテグリンに結合し、それによって細胞内アクチン束とECMの間に機械的結合を形成します[24]。したがって、次に、接着斑複合体の成分の発現を分析した。結果は、VCLとFAKのタンパク質とmRNAの発現がF-アクチンの変化と一致していることを示し、接着斑複合体がTiO 2 によって誘導されるMSCの骨形成分化のプロセスに関与していることを示しています ナノチューブ(図5bおよび6a、b)。さらに、GTPaseのRhoファミリーに含まれる低分子量GTPaseタンパク質であるRhoAが、Jasp処理グループでアップレギュレーションされ、Cyto Dによって阻害されることもわかりました(図5bおよび6a、b)。 RhoAはMAPK経路の重要な上流シグナル伝達分子であり、FAKによって調節される可能性があります[25、26]。 RhoAの主な機能は、ストレスファイバー(F-アクチン)の重合と安定性、および接着斑複合体の集合を促進することです[27]。まとめると、これらのデータは、TiO 2 ナノチューブは、フォーカルアドヒージョンコンプレックスとRhoAを介してF-アクチン重合に影響を与える可能性があります。
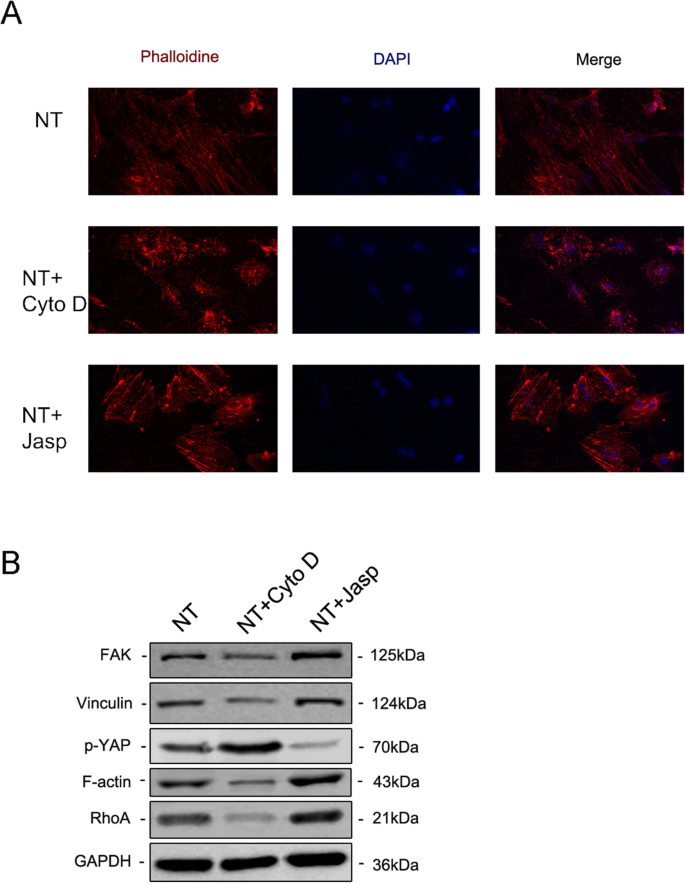
免疫蛍光染色により、ローダミン結合ファロイジン( a )で染色することにより、F-アクチンのレベルが明らかになりました。 )。 The protein expression of FAK and VCL contained in the focal adhesion complex, RhoA and phosphorylated YAP were investigated by Western blotting (b )。 NT the nanotubes group
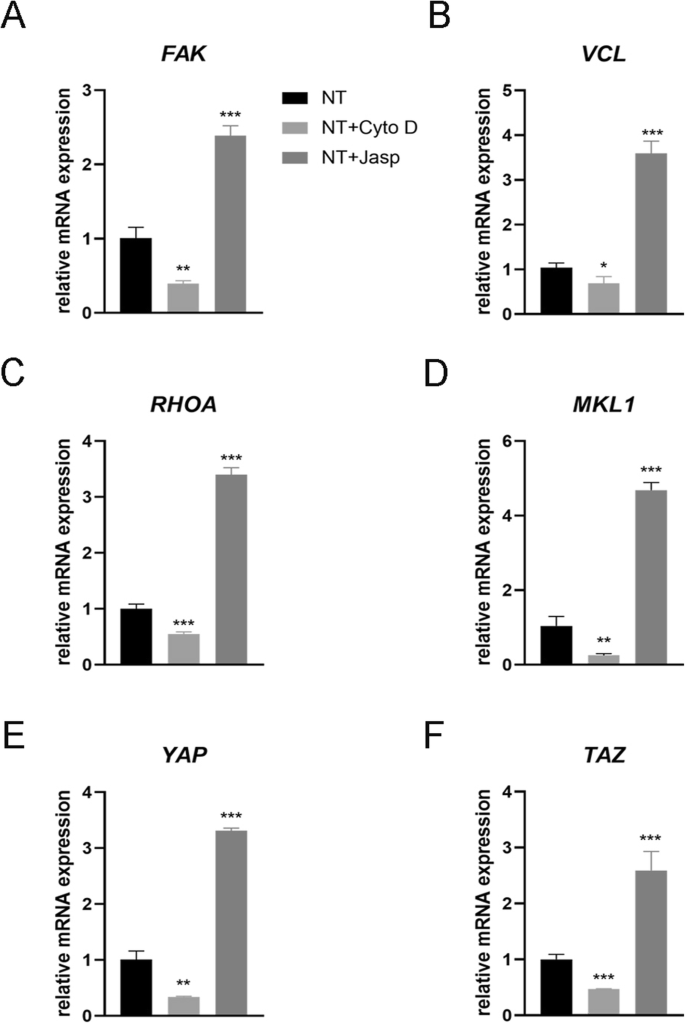
Effect of F-actin assembly on FAK (a ), vinculin (VCL) (b ), RhoA (c ), MKL1 (d ), YAP (e ), and TAZ (f ) gene expression in MSCs. NT the nanotubes group. Data represent the mean ± SD of three samples. * P <0.05、** P <0.01, and ***P <0.001
But how does F-actin regulate cell fate? Most studies have demonstrated that F-actin is involved in cell migration, cell division, endocytosis, and especially tumor cell invasion [28,29,30]. Few studies have suggested that F-actin could also regulate cell differentiation, let alone its specific molecular mechanism [31, 32]. Consequently, we searched for articles that mentioned the F-actin changes and found that YAP/TAZ, two closely related transcriptional co-activators in the Hippo signaling pathway, which shuttle between the cytoplasm and the nucleus, may serve as mechanotransducers in regulating MSC differentiation [33,34,35]. In addition, we also found that MKL1, a key regulator of smooth muscle cell differentiation, which interacts with the transcription factor serum response factor, could bind to G-actin and also circulate between the cytoplasm and the nucleus [21, 36]. Our results ultimately identified the involvement of YAP/TAZ and MKL1 in nanotube-induced osteoblast differentiation mediated by F-actin (Figs. 5b and 6d–f). Interestingly, the protein expression of phosphorylated YAP showed the opposite trend, indicating that not only was the expression of YAP changed, but the phosphorylation of YAP was also changed by Cyto D and Jasp (Fig. 5b). This result was consistent with the report that the phosphorylation of YAP/TAZ could be sequestrated in the cytoplasm [35].
In summary, our results preliminarily demonstrated that F-actin regulated osteoblast differentiation of MSCs on TiO2 nanotubes through MKL1 and YAP/TAZ (Fig. 7).
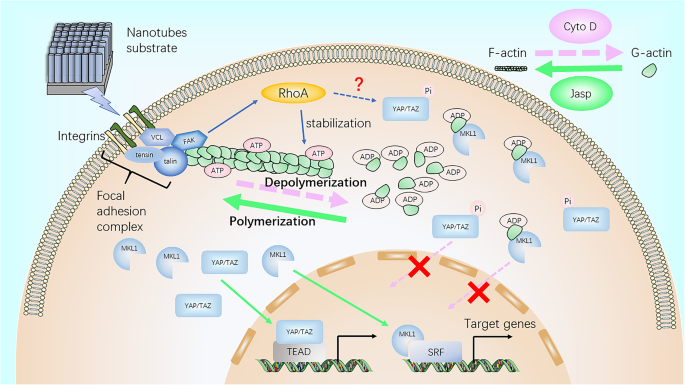
Schematic representation of F-actin assembly induced by nanotubes, and the putative role of MKL1 and YAP/TAZ in acting as the downstream mediators of F-actin signaling to regulate gene expression
ディスカッション
Titanium and titanium alloys are the most widely used metal materials in orthopedic clinical implants due to the good properties of titanium [3]. However, aseptic loosening is still an urgent problem to be solved and improved, and the key is likely to lie in improving the integration of the implant and the host bone. Previous studies have shown that surface coating and modification or immobilization of biofunctional molecules will be beneficial to osseointegration [37]. Recently, the surface topography of implants has attracted the attention of many researchers thanks to studies into the cell response to physical cues [9,10,11, 13, 38]. In this study, we demonstrated the ability of nanotubes to promote osteogenic differentiation of MSCs, and this ability was enhanced with increasing inner diameter of the nanotubes (30–70 V). This will help guide the diameter of the nanotubes on the surface of the implants.
As a topographical structure, nanotubes first change the physical properties of the material, such as adsorption capacity and electrical and thermal conductivity. These physical properties determine their application in the industrial field. For example, most high-voltage power transformers need to be filled with insulating material, which is usually transformer oil or insulating gas. When the insulation of a transformer fails due to overheating and partial discharge, a serious discharge accident will occur. Therefore, finding an effective method that accurately detects the concentration and types of dissolved gases or insulating gas decomposition components in a transformer is necessary to monitor the operating state of the transformer [8, 39,40,41]. The traditional approach is to look for materials with good gas adsorption in transition elements, which are rich in d electrons, such as Pd(1 1 1) [39]. Nowadays, nanotubes are widely studied for their good gas adsorption properties.彼等。 found that CuO-BNNT was suitable for the adsorption of C2 H 2 , because of its stronger adsorption on C2 H 2 [8]. Meanwhile, TiO2 itself can be a gas-sensing material. Gui et al. found that Co-doped TiO2 further enhanced gas adsorption capacity and exhibited a superior adsorption ability and conductivity change toward C2 H 4 molecules [40]. Consistent with this study, Mn-doped graphene also exhibited enhanced conductivity and superior capability of C2 H 2 and CO detection than pristine graphene [41]. The above research indicates that the TiO2 nanotubes prepared in our experiment have a potential application in the field of monitoring the operative state of a transformer. However, the adsorption capacity and electrical conductivity of the nanotubes to gases need to be further studied, especially whether these properties are enhanced after doping with transition elements (e.g., Mn).
In addition to changing the physical properties of a surface, nanoscale morphology also affects the biological behavior of the cells attached to it. Cells first adhere to the surface of the material and then migrate, proliferate, and differentiate. Compared to a flat surface, the hollow structure of the nanotubes provides fewer adhesion sites for cells. Therefore, in order for the cells to adhere to the nanotube surface steadily and maintain the biomechanical balance within the cell, the focal adhesion complex begins to assemble and mature, and F-actin becomes strong and stable.
F-actin, a linear polymer microfilament consisting of G-actin monomers, is one of the three major components of the cytoskeleton. As a mechanical-loading structure, F-actin is generally believed to be involved in cell division, cell migration, endocytosis, and tumor cell invasion [28,29,30], but some recent studies showed that it can also affect cell differentiation [31,32,33, 36]. For example, actin cytoskeletal depolymerization by simvastatin induces chondrocyte differentiation [31], and actin depolymerization enhances adipogenic differentiation in human stromal stem cells [32]. Our results also revealed that, compared with the control group, MSCs cultured on nanotubes had higher F-actin levels and a more obvious fibrous structure. Meanwhile, promotion of F-actin polymerization by Jasp enhanced osteogenic differentiation, while the depolymerization of F-actin inhibited osteogenic differentiation, suggesting that F-actin mediates TiO2 nanotube-induced osteoblastic differentiation of MSCs.
F-actin can be regulated by Rho GTPases, members of the Ras superfamily [23, 42], and Rho can induce actin reorganization through at least two effectors, ROCK and Dia. ROCK is activated by binding to Rho-GTP and then myosin light chain (MLC), the substrate of ROCK, plays an important role in F-actin assembly. ROCK inhibits the activity of MLC phosphatase, leading to an increase in MLC phosphorylation, which stimulates the ATPase activity of myosin II and promotes the assembly of F-actin. In addition, ROCK also targets LIM kinase (LIMK). Phosphorylated LIMK inactivates cofilin by phosphorylation, which can disassemble F-actin in its active state. Another effector is Dia, a member of the formin-homology (FH) family of proteins which contains two FH domains. These domains contain multiple proline-rich motifs which bind to the G-actin-binding protein, profilin. This interaction contributes to actin polymerization and F-actin organization [42]. We detected one of the Rho GTPases, RhoA, and found that the expression of RhoA was consistent with the level of F-actin. However, we were unable to clearly describe how the nanotubes regulate the expression of RhoA, because there are many other regulators, including integrin signaling, other adhesion receptors, G protein-coupled receptors (GPCRs), soluble factors such as LPA, receptor tyrosine kinase signaling, and so on [43].
Knowing that F-actin can be regulated by RhoA, we next asked what role focal adhesion played in this process, because focal adhesion complexes, containing integrins, talin, vinculin, paxillin, and focal adhesion kinase (FAK), are formed and mature when cells attach to the surface of nanotubes. Integrins are transmembrane heterodimers that couple the ECM to the other focal adhesion proteins so as to facilitate cell attachment. They not only act simply as hooks but also transmit to the cell critical signals about the nature of its surroundings, which along with other signals such as EGFR, prompt the cell to make decisions about its biological behaviors. These signals are further transmitted to F-actin, which is directly connected to the focal adhesion complexes. On the one hand, the nanoscale morphology causes focal adhesion complex assembly and maturation. On the other hand, kinases such as FAK and Src kinase family members will recruit molecules such as CRK to self-regulate the assembly and maturation of focal adhesion complexes [44,45,46]. Our results demonstrated that the formation and maturation of focal adhesion complexes were impaired by F-actin depolymerization, suggesting that there was a feedback from focal adhesion complexes to actin assembly in line with published reports.
However, it should not be ignored that these proteins contained in focal adhesion complexes have the function of signal transduction [47]. That is to say, nanotubes may directly regulate gene expression through signal cascades, and F-actin may just participate in or be affected by this process. For instance, the dual kinase complex of FAK and Src can regulate Rho GTPases such as RhoA. This shows that nanotubes can regulate RhoA through integrins and the FAK/Src complex. In addition Src, a non-receptor tyrosine kinase protein, can activate Ras (small GTPase) by phosphorylating FAK at tyrosine residue 925 [47, 48]. Then, Ras activates numerous biochemical pathways, including the well-studied MAPK pathway and the PI3K/AKT/mTOR pathway. In the MAPK pathway, Ras activates c-Raf, followed by mitogen-activated protein kinase kinase (MAP2K) and then MAPK1/2, also known as extracellular signal-regulated kinase (ERK). ERK in turn activates transcription factors such as serum response factor (SRF) and c-Myc that are involved in regulating growth and differentiation [49]. What is more, Runx2, a key transcription factor in osteogenic differentiation, can also be regulated by ERK [50], and our previous study confirmed that mechanical strain promoted osteogenic differentiation of BMSCs through the FAK-Erk1/2-Runx2 pathway [17]. Therefore, we cannot rule out that ERK plays a role in nanotube-induced osteogenic differentiation and further study is still needed.
So what exactly is the role of F-actin in inducing differentiation of nanotubes, because its change can affect cell differentiation? One possibility is that the change of F-actin assembly can inversely regulate the level of FAK so as to induce osteogenic differentiation through the FAK-Erk1/2-Runx2 pathway as described above, because in our results, focal adhesion complexes and actin polymerization showed the same trends of change, indicating that they act as a whole in response to the extracellular environment. However, some other possibilities also exist, and a number of articles have shown that MKL1 and YAP/TAZ act downstream of the actin dynamic balance [20, 51,52,53,54]. Both of them shuttle between the cytoplasm and the nucleus, and may help to transduce signals from the cytoskeleton to the nucleus.
MKL1, also termed myocardin-related transcription factor A, is sensitive to changes in G-actin levels. When cytoplasmic G-actin levels increase, monomeric G-actin binds to MKL1 and prevents it from binding to SRF and activating transcription. SRF target genes include actins such as smooth muscle actin (SMA) as well as other actin-binding proteins, including immediate early genes like c-fos and egr1. Recent studies have demonstrated that changing SRF activity could regulate adipogenesis by activating the adipogenesis transcription factor peroxisome proliferator-activated receptor γ (PPARγ), and also regulate bone formation via IGF-1 and Runx2 signaling [55, 56].
YAP and TAZ are two transcriptional coactivators in the Hippo signaling pathway, identified as an important regulatory pathway that restricts cell proliferation, thereby controlling organ size and morphogenesis [20]. Large tumor suppressor genes 1 and 2 (LATS1/2) phosphorylate them, thereby creating a binding site for 14-3-3 proteins, the binding of which prevents their nuclear import [53, 54]. As a consequence, phosphorylated forms of YAP/TAZ are sequestered in the cytoplasm, preventing the expression of genes like Ctgf and Areg. In addition, some studies have shown that YAP/TAZ can interact with T-box 5 (TBX5), RUNX2, and p73 to regulate gene expression [57,58,59]. Further, cell adhesion to cell matrix proteins has been shown to trigger YAP nuclear localization through an integrin/FAK/Src axis. In our study, the results suggested that this pathway was possibly involved in nanotube-induced differentiation. Further study into the downstream mediators of the integrin/FAK/Src axis should be carried out to clarify the specific mechanism.
On the other hand, more and more studies illustrate that F-actin interacts with Hippo signaling, and somehow inhibits the phosphorylation of YAP [54, 60], which is consistent with our experimental results that promoting F-actin polymerization reduces the expression of phosphorylated YAP. We hypothesize that ATP involved in the process of the transformation between G-actin and F-actin may also play an important role in the phosphorylation of YAP, which is yet to be studied.
After understanding the above possible molecular mechanisms, we can try to explain some of the experimental phenomena found in this study. Our results revealed that the larger the diameter of the nanotubes, the stronger the ability of the nanotubes to promote osteogenic differentiation. This is consistent with previous research [61, 62]. The reason for this phenomenon is that the larger the diameter of the nanotubes, the less adhesion sites they can provide to the cells, and the greater the assembly and maturity of focal adhesion complexes. Along with these, stress fibers made of F-actin will have greater strength and stability. These structures enhance the signaling that promotes osteogenic differentiation. Predictably, however, this effect is significantly reduced when the nanotubes become too large in diameter, making it difficult for the cells to adhere to the surface [12]. Similarly, when the height of the nanotubes is inconsistent, the differences in height can result in a change of adhesion site and rearrangement of the cytoskeleton, which will further affect cell differentiation. Intriguingly, even flat surface materials without nanotube modification can induce changes in cell differentiation. A number of studies have demonstrated that focal adhesion formation and stress fiber organization are regulated by substrate stiffness [63,64,65], and YAP/TAZ also plays an important role in this process. Therefore, it is obvious that the integrins–FAs (focal adhesions)–F-actin axis plays a role in the transduction of physical signals into intracellular chemical signals.
In summary, our results demonstrated that F-actin regulates osteoblastic differentiation of mesenchymal stem cells on TiO2 nanotubes through MKL1 and YAP/TAZ, whose target genes partly explained the proliferation and differentiation of MSCs. We know that there is no single change in the signal network and any change is regulated by numerous molecules and proteins. One type of biological behavior must be the result of the regulation of a series of signaling pathways. Nanotubes induce cell differentiation by triggering a complex network of signals, including integrins, proteins contained in focal adhesion complexes, FAK, Src, Rho GTPase, the MAPK pathway, the Hippo pathway, and other reported signaling pathways. At least as important, there are many signal cycles in the signal network and a downstream signal can regulate the upstream signal via feedback. In this study, we found that vinculin and FAK can be regulated backwards by F-actin assembly, increasing the uncertainty of molecular function. Therefore, more details of the molecular mechanism await further study.
結論
Our results showed that TiO2 nanotubes promoted the osteogenic differentiation of MSCs, and this ability was enhanced with the increasing diameter of nanotubes within a certain range (30–70 V). F-actin mediated nanotube-induced cell differentiation through MKL1 and YAP/TAZ, providing a novel insight into the study of cell differentiation.
データと資料の可用性
The datasets used and analyzed during the current study are available from the corresponding author on reasonable request.
略語
- MSCs:
-
Mesenchymal stem cells
- SEM:
-
走査型電子顕微鏡
- EDS:
-
X-ray energy dispersive analysis
- AFM:
-
原子間力顕微鏡
- ALP:
-
アルカリホスファターゼ
- Cyto D:
-
Cytochalasin D
- Jasp:
-
Jasplakinolide
- VCL:
-
Vinculin
- FAK:
-
Focal adhesion kinase
- BCA:
-
ビシンコニン酸
- PVDF:
-
Polyvinylidenedifluoride
- Runx2:
-
Runt-related transcription factor 2
- Osx:
-
Osterix
- OCN:
-
Osteocalcin
- YAP:
-
Yes-associated protein
- MKL1:
-
Megakaryoblastic leukemia 1
- FBS:
-
ウシ胎児血清
- ECM:
-
Extracellular matrix
- MLC:
-
Myosin light chain
- LIMK:
-
LIM kinase
- FH:
-
Formin-homology
- GPCR:
-
G protein-coupled receptors
- MAP2K:
-
Mitogen-activated protein kinase kinase
- ERK:
-
Extracellular signal-regulated kinase
- SRF:
-
Serum response factor
- SMA:
-
Smooth muscle actin
- PPARγ:
-
Peroxisome proliferator-activated receptor γ
- LATS1/2:
-
Large tumor suppressor gene 1 and 2
- TBX5:
-
T-box 5
ナノマテリアル
- 二酸化チタン-TiO2-価格、市場および分析
- TiO2ナノ流体に向けて—パート1:準備と特性
- ナノテクニックは癌幹細胞を不活性化する
- TiO2ナノ流体に向けて—パート2:アプリケーションと課題
- 色素増感太陽電池の光学的および電気的特性に及ぼすTiO2中の金ナノ粒子分布の影響
- ZnOナノ結晶の合成と逆ポリマー太陽電池への応用
- ペロブスカイト太陽電池用のTiO2コンパクト層を製造するための最適なチタン前駆体
- ナノ粒子の跳ね返りに及ぼす弾性剛性と表面接着の影響
- ペロブスカイト太陽電池の変換効率に及ぼすTiO2コンパクト層に埋め込まれた異なるサイズと濃度のAgナノ粒子の影響
- Ho3 + -Yb3 + -Mg2 +トリドープTiO2の新しいアップコンバージョン材料とそのペロブスカイト太陽電池への応用
- カーボンナノチューブとその誘導体がinvitroでの腫瘍細胞および生化学的パラメーター、invivoでの細胞血液組成に及ぼす影響