ナノ粒子:癌の診断と治療をアップグレードするための新しいアプローチ
要約
従来の癌治療法は、さまざまな副作用と標的腫瘍への不十分な損傷のために批判されてきました。ナノ粒子の飛躍的進歩は、従来の治療と診断をアップグレードするための新しいアプローチを提供します。実際、ナノ粒子は、従来の癌の診断と治療の欠点を解決するだけでなく、腫瘍の診断と治療のためのまったく新しい視点と最先端のデバイスを生み出すこともできます。ただし、ナノ粒子に関する研究のほとんどはinvivoおよびinvitroの段階にとどまり、ナノ粒子に関する臨床研究はほとんど報告されていません。このレビューでは、最初に癌の診断と治療におけるナノ粒子の現在のアプリケーションを要約します。その後、NPの臨床応用を妨げる課題を提案し、過去2年間に更新された文献と組み合わせて実行可能なソリューションを提供します。最後に、腫瘍の診断と治療におけるNPの今後の展開について意見を述べます。
はじめに
腫瘍の発生率と死亡率は世界中で高いままです。毎年、1400万人近くの新しい癌患者がおり、800万人が癌関連疾患で亡くなっています[1]。近年、化学療法、標的療法、放射線療法、外科手術などの従来の腫瘍治療は、進行中の行き詰まり、多くの副作用および満足できない治療結果について絶えず批判されています。従来の腫瘍治療法の欠点のために、標的能力、効果的な腫瘍幹細胞殺傷能力、および軽微な副作用を備えた新しい腫瘍医療法を模索する研究がますます増えています。新しい腫瘍治療法には、免疫療法、標的療法、物理的切除、遺伝子治療、光力学療法(PDT)、および光熱療法(PTT)が含まれますが、これらは従来の腫瘍療法と比較して優れた効果を示しています。ここでの処理方法はすべて、キャリアの協力を必要とする共通の特徴を持っています。ウイルスはキャリアとして使用できますが、ウイルスベクターは挿入型遺伝子変異と免疫原性を引き起こすことが確認されています[2]。したがって、より安全で効果的なキャリアを見つけることが最優先事項になっています。
ナノ粒子のサイズが小さいため、バイオセーフティ、薬物負荷、および物理的特性が理学療法を支援できるため、ナノ粒子は新しい腫瘍治療法の担体としてますます利用されています。これらのナノ粒子を介した治療法は、多機能で、副作用が少なく、治療効果が高いという長所があります[3]。さらに、ナノ粒子によって媒介される多くの医用画像技術は、より優れた明瞭さと精度を備えており、正確な腫瘍診断に役立ちます[4]。ナノテクノロジーと医療技術の発展に伴い、金、銀、鉄、リポソームなどの金属や生物学的材料が医療用ナノ粒子(NP)の製造に広く適用されています[5]。現在、多くの研究者は、物理的、化学的、および/または生物学的特性に基づいてこれらの材料を利用して、薬物、造影剤、さらには遺伝子をナノ粒子に埋め込み、薬物標的化送達、強化されたイメージング、凍結手術、PTTおよびPDT [6]。
さらに、ほとんどのナノ粒子がinvivoおよびinvitroの段階でしか留まらないという現象があります。ただし、NPの臨床応用を思いとどまらせる理由を要約する文献が不足しています。したがって、この記事は、腫瘍の診断と治療の分野におけるナノ粒子の適用状況を要約するだけでなく、臨床応用へのナノ粒子の侵入を阻害する要因を見つけ、実行可能な解決策を提案することも目的としています。
医療用機能性ナノ粒子の調製と特性評価
医学で一般的に使用されるナノ粒子は、その構成材料や機能に応じて、金属ナノ粒子、非金属ナノ粒子、複合ナノ粒子の3種類に分類でき、物理的および化学的性質はサイズや形状などのパラメーターの影響を受けます。したがって、さまざまな適用方向でのナノ粒子の機能要件を考慮すると、適切な調製プロセスを選択することが非常に重要です。ナノ粒子のすべての調製方法は、ボトムアップアプローチとトップダウンアプローチの2つの方法に分類できます。ボトムアップアプローチは基本的に、ナノ粒子を形成するために互いに積み重ねられた基本ユニット(原子、分子、さらに小さな粒子を必要なナノ構造を組み立てるための基礎として使用できます)を介して行われますが、トップダウンアプローチは基本的に完全な固体材料ですナノ粒子に分解し始めます[7]。表1に、医療用ナノ粒子の調製例をいくつか示します。
<図> 図>医学で一般的に使用される3種類のナノ粒子の中で、金属ナノ粒子が最も広く使用されています。金属ナノ粒子材料には、金属および金属酸化物が含まれる。金属ナノ粒子の最も一般的に使用される調製プロセスは、日本の科学者杉本らによって提案されたゾルゲル(Sol-Gel)プロセスです。 1990年代には、液相で単分散金属酸化物粒子を調製するためによく使用されます。ゾルゲル法はボトムアップの準備プロセスです。金属ナノ粒子を調製するためのこの方法の主な原理は、化学的および物理的手段によって金属イオンの均一に分散したゾルを形成し、次にレドックス反応によってゲルを形成することです。ゲルで生成された金属ナノ粒子は、制御可能に核形成、成長、堆積することができます。実験に使用した金属コロイドの単分散性、金属イオンと酸化剤・還元剤の濃度関係が制御されている限り、合成された金属ナノ粒子のサイズを制御することができます。図1は、ゾルゲル法の概略図です。
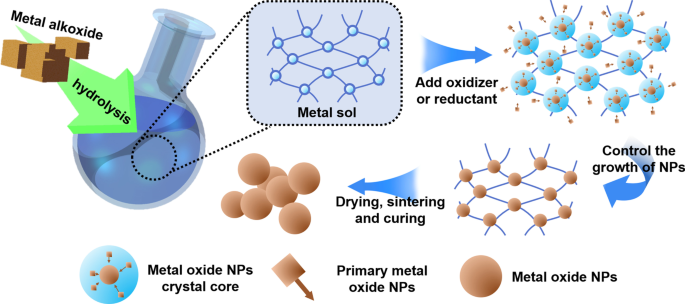
ゾルゲル法の概略図
金属ナノ粒子を調製するために一般的に使用されるボトムアップ法には、共沈法、熱水アプローチ、および光化学的方法が含まれます。共蒸着法は、液体環境での核形成、成長、凝集を同時に行うプロセスです。溶液が過飽和になると、多数の小さなサイズの粒子不溶性生成物が得られます[15]。水熱法は、溶液中の材料に適用される蒸気圧を制御することにより、得られるナノ粒子の形態を制御するために液体環境で実行されるプロセスです。さらに、電線の爆発やボールミルなど、金属ナノ粒子を調製するためのトップダウンの方法がいくつかあります。電線爆発の原理は、電気爆発の過程で、金属原子が蒸発し、電解質中で急速に冷却されて酸化物ナノ粒子を形成することです。電解質の組成と電流強度を制御することにより、より微細で均一なナノ粒子を制御できます。ボールミルは、適切な研削時間と関連する機器のプロセスパラメータを選択することにより、遊星歯車のフライス盤などの工作機械を使用して、サイズを制御できるナノ粒子を迅速かつ大規模に製造する方法です。金属ナノ粒子に加えて、この調製方法は他の種類のナノ粒子にも適用できます。
2番目の一般的なタイプは非金属ナノ粒子です。医学で一般的に使用される非金属ナノ粒子には、ポリマーナノ粒子、生体分子由来のNP、炭素ベースのNP、およびシリカナノ粒子が含まれます[16、17、18]。その中で、シリカナノ粒子が最も代表的です。シリカ表面には豊富なヒドロキシル基があり、表面でのプローブまたは蛍光基の結合を促進するため、より柔軟な機能を備えています。シリカナノ粒子の一般的に使用される合成方法は、ゾルゲル法とストーバー法です[19、20]。古典的なストーバー法は、アルカリ性条件下でのケイ酸塩の加水分解と凝縮によるシリカナノ粒子の簡単で効率的な調製です。
ナノテクノロジーの発展に伴い、複合ナノ粒子はその優れた機能的適合性のために開発されてきました。金属ナノ粒子は、プラズモン共鳴効果(SPR)や磁場での制御性など、非金属ナノ粒子にはない多くの特性を持っていますが、金属粒子は体内で効果的に分解することが難しく、過度の使用には一定の毒性があります細胞へ[21]。したがって、異なる材料のナノ粒子を異なる調製方法で複合ナノ粒子に組み合わせると、機能拡張を実現できます。魏ら。金ナノロッド(Au NR)を調製し、Au NR上でN-イソプロピルアクリルアミド(NIPAAM)の表面開始原子移動ラジカル重合(SI-ATRP)を実行して、近赤外応答ナノハイブリッドを合成しました[22]。金属とポリマー材料を組み合わせたこの複合ナノ粒子は、光熱と近赤外光の両方に対応する薬物放出能力を備えています。包み込むヒドロゲルシェルにより、このナノ粒子は単一のAuナノ粒子よりも優れた生体適合性を示します。 Prakashは、コアとしてAuを使用し、SiO 2 を使用して複合NPを合成しました。 改良されたストーバー法によるシェルとして。コアシェルナノ粒子の不活性シェルは、金属粒子の毒性を低減し、元の単一金属NPの材料安定性と薬物運搬能力を向上させるのに有益です[23]。
上記のナノ粒子の従来の調製方法に加えて、ナノテクノロジー科学の発展に伴い、生態学的および環境保護の新しい要件が提唱され、新しい環境に優しいナノ粒子合成方法が出現しました[24]。初めて、Hajar等。 Stevia rebaudianaを生物学的還元剤として使用して、粒子サイズが1〜40nmの範囲のZnSナノ粒子を正常に合成しました。このように合成されたZnSナノ粒子は優れた生体適合性を持っています[25]。グリーンケミストリーの原則によれば、Miri etal。 P. farcta(マメ科に属する植物)抽出物を使用して、CeO 2 をすばやく合成しました 粒子サイズが約30nmのNP。この種のナノ粒子は、優れた生体適合性を備えています[26]。
医用画像用ナノ粒子
医用画像は、腫瘍の診断と治療において重要な役割を果たします。酸化鉄NPのような多くのナノ粒子は、イメージングを強化できる光学的、磁気的、音響的、および構造的特性を備えています(図2)。いくつかの研究は、標的組織にNPを導入することで、画像のコントラストを改善し、腫瘍の手術と診断のためのより良い画像ガイダンスを提供できることを示しています[27]。たとえば、凍結手術では、NPは腫瘍とアイスボールのエッジの画像品質を向上させることができ、アイスボールを正確に覆い、治療効果を向上させるのに役立ちます[28]。さらに、イメージングで使用されるナノ粒子のほとんどは金属でできています。異なるイメージング原理の違いによると、ナノ粒子も異なる金属材料でできています。表2に、医用画像用のさまざまな材料で作られたNPに関する最近の例をいくつか示します。
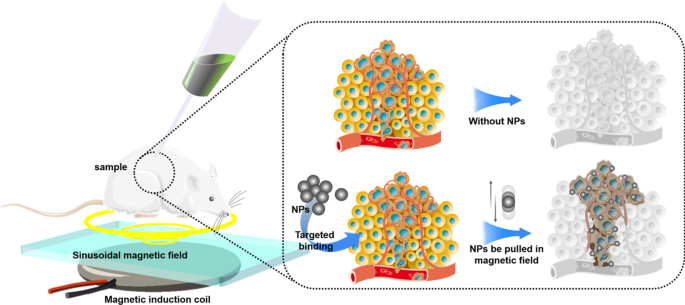
NPの改善されたイメージングの図解
光コヒーレンストモグラフィー(OCT)は、非侵襲的なミクロンレベルの解像度および生物医学画像技術です。 OCTは、リアルタイムの診断と外科的ガイダンスに役立ちます。ただし、OCTは非弾性散乱光を検出できません。これは、この光が入射フィールドでコヒーレントではないためです[35]。最近、多くの研究により、NPの運動状態がOCTの振幅を変化させることができることが証明されており、これがこの問題に対処している可能性があります。磁場を介したNPの移動を妨げると、光散乱に局所的な変化が生じる可能性があります。いくつかの研究では、磁場に磁気NPを配置してその動きを制御すると、その領域の光散乱が変化する可能性があるため、元々インコヒーレントな非弾性散乱光を検出できることが指摘されています。この新しいイメージング方法は、起磁力光コヒーレンストモグラフィー(MMOCT)[36]です。
MRIは、最も効果的な非侵襲的腫瘍検出技術の1つです。それにもかかわらず、生物学的背景と癌組織との間のMRI信号比較の欠如は、しばしば臨床腫瘍診断に影響を及ぼします[37]。 MRIは、水分子中の水素分子の磁化を測定するスキャニングイメージング法です。各組織の陽子が磁化に異なる変化を引き起こすため、各解剖学的構造は異なる画像を提示します。より多くの造影剤を適用することにより、画像の視認性を向上させることができます[38、39]。腫瘍の早期発見に広く利用されている腫瘍関連EPR効果は、磁性NPに対して優れたコントラスト増強能力を生み出します[40]。現在最も一般的なMRIナノプローブ造影剤である酸化鉄磁気NP(IONP)は、特定の細胞ターゲティングを持っています[41]。たとえば、研究によると、IONPはMRIを使用して肝臓癌の診断中に健康な肝臓クッパー細胞に入ることができますが、癌細胞から除外され、低信号の健康組織と高信号の腫瘍組織が得られます[42]。最近の研究に基づいて、適切な粒子表面修飾と適切な腫瘍特異的バイオオリゴマーのNP埋め込みは、腫瘍内のNPをより適切に固定して、より明確なイメージング結果を達成し、初期の微小腫瘍イメージングにも使用できます。たとえば、研究によると、ヒトトランスフェリンを標的とするAuNPは、脳腫瘍の画像効果を大幅に高めることができます[43]。 Gao etal。常磁性NPプローブに基づく抗上皮成長因子受容体モノクローナル抗体(mAb)を装備し、小さな腫瘍の画像化を実現します[44]。
標的化ドラッグデリバリー用のナノ粒子
化学療法薬は現在、腫瘍に対して最も一般的に使用されている治療法ですが、それでも、悪性腫瘍領域での標的濃縮が不十分であり、健康な組織で過剰に蓄積するという問題があります[45]。これは、骨髄、毛包、胃腸細胞、リンパ球などの活発に分裂する細胞の阻害を引き起こし、骨髄抑制、粘膜炎、脱毛、さらには死などの有害反応を引き起こす可能性があります[46]。薬物送達のための正常細胞と癌細胞の能動的分化を指す標的化薬物送達は、従来の治療よりも優れた有効性と少ない副作用を示します[45]。多くの研究により、NPは能動的または受動的標的化を通じて化学療法薬を腫瘍細胞に標的化できることが確認されています[47]。さらに、多くの実験により、NPは免疫抑制剤の標的化送達においても重要な役割を果たすことがわかっています[48]。
図3に示すように、パッシブターゲティングは、異常な血管、温度、pH、腫瘍細胞の表面電荷など、腫瘍組織の病理生理学的特性に依存することがよくあります[49]。たとえば、強化された透過性と保持効果(EPR )腫瘍組織の血管のうち、直径が約400nmのNPを腫瘍組織に受動的に移行させることができます[50]。ただし、直径、表面電荷、分子量、疎水性、親水性などのNPの物理化学的特性に関して、パッシブターゲティングアプローチには多くの制限があります。その上、受動的ターゲティング技術は、薬物拡散効率が不十分であり、腫瘍細胞において不十分なEPR効果を示します[51]。パッシブターゲティングの欠陥により、近年、ドラッグデリバリーNPに関するほとんどの研究はアクティブターゲティング(リガンドターゲティング)にシフトしています。表3は、ドラッグデリバリーで使用されるNPに関する最近の例を示しています。
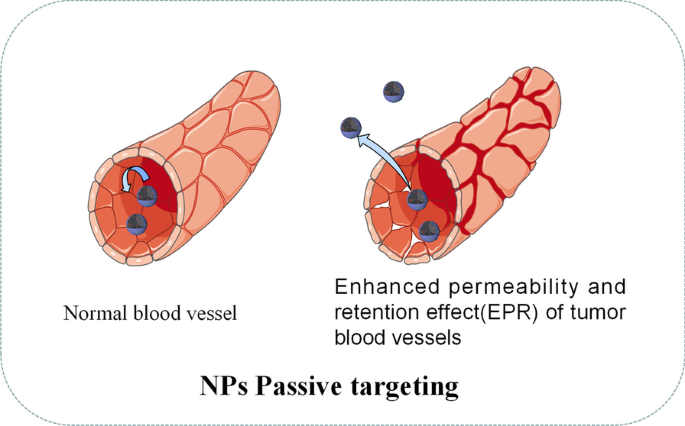
NPのパッシブターゲティングの図解
アクティブターゲティング(リガンドターゲティング)NPは、腫瘍特異的バイオマーカーのいくつかのリガンドを運ぶことがよくあります[61]。図4に示すように、リガンドが腫瘍表面の受容体に接触すると、NPは受容体を介したエンドサイトーシスによって腫瘍細胞に取り込まれ、酸性pHと細胞内環境の特定の酵素によって薬物が放出されます[ 62]。標的リガンドに関しては、葉酸、トランスフェリン、上皮成長因子受容体(EGFR)および糖タンパク質が現在の研究で一般的に利用されています[62]。たとえば、Sandoval etal。ゲムシタビンを備えたEGFR標的ステアリルNPを介した乳がんのマウスの治療において、有意な薬物濃縮と明らかな有効性が観察されました[63]。 Pandey etal。塩酸ベルベリン(BHC)を運ぶ葉酸を標的とした金のNPは、葉酸受容体を発現するヒト子宮頸がん細胞に効果的に薬物を送達できることを発見しました[64]。
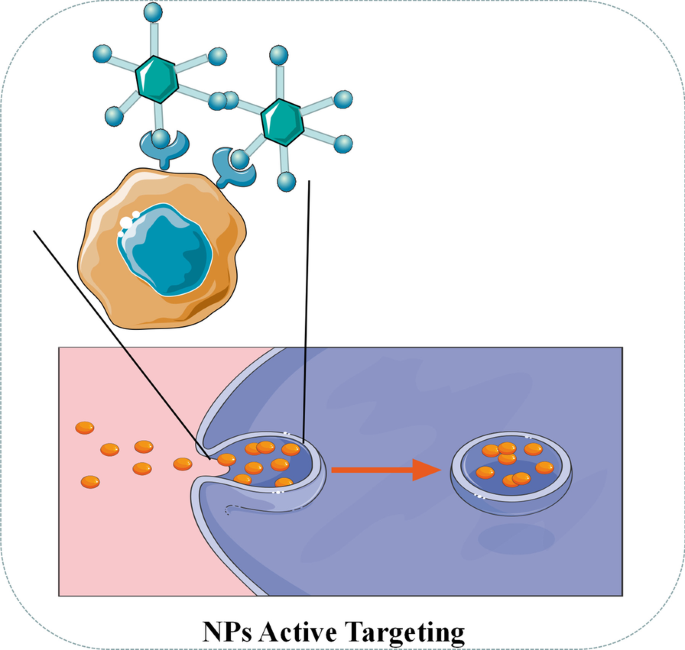
NPのアクティブターゲティングの図解
近年、化学療法薬と比較して、短鎖干渉RNA(siRNA)を介した遺伝子サイレンシング療法が腫瘍治療の新たな展望と見なされています[64]。ウイルスはsiRNAの送達媒体として使用できますが、ウイルスベクターは挿入型遺伝子変異と免疫原性を引き起こすことが確認されています[65]。対照的に、微量元素のセレン自体が腫瘍の発生を減らし、薬物毒性を低下させ、免疫機能を調節できるため、セレンNPはsiRNAキャリアとして大きな可能性を秘めていると報告されています[66]。さらに、セレンNPの表面は、さまざまな腫瘍標的化部分(葉酸、ヒアルロン酸、RGDペプチドなど)をロードして、腫瘍標的化能力を高めることができます[67]。 Xia etal。 RGDfCペプチドの標的となるセレンNP(RGDfC-Se @ siRNA)は、HeLa子宮頸がんを標的とする優れた能力を持っていると報告されています[60]。一方、RGDfCはα v と具体的に組み合わせることができるため β 3 さまざまな腫瘍細胞によって高度に発現されるインテグリンであるRGDfC-Se @ siRNA NPは、さまざまな腫瘍の標的化ドラッグデリバリーに再利用できます[68]。構造に関しては、正電荷を持つRGDfC-SeNPは、静電相互作用によって負電荷のsiRNAをしっかりとパッケージ化できます[69]。動物実験を通じて、RGDfC-Se @ siRNA NPは、クラスリン関連エンドサイトーシスを介して腫瘍細胞に効率的に侵入する能力を示しています。腫瘍細胞では、siRNAを迅速に放出し、関連遺伝子を効率的にサイレンシングし、活性酸素種(ROS)の生成を促進して、腫瘍細胞の増殖を阻害し、腫瘍細胞のアポトーシスを促進します[69]。さらに、複数のSeNPは優れた生物学的安全性を示しており、肝臓、腎臓、心臓、肺、脾臓、その他のマウスの主要臓器に明らかな毒性損傷はありません[60、70、71]。
現在、標的化ドラッグデリバリーで使用される多くのNPがありますが、ほとんどのアプリケーションはまだ細胞または動物実験の段階にあり、強力な臨床アプリケーションのサポートが不足しています。さらに、多くのNPは腫瘍内に投与されるため、腫瘍に適用できるNPの範囲が制限され、特別なNPドラッグデリバリーツールやその他のドラッグデリバリー方法がありません。
したがって、NPを投与するためのより良い方法を探求することは、標的化ドラッグデリバリーNPの将来の研究の方向性となる可能性があります。既存の学術雑誌によると、血管インターベンション投与は実行可能な方法である可能性があります。仮定では、最初にイメージングの助けを借りて腫瘍供給血管の位置を特定し、次にガイドワイヤーを使用して腫瘍供給血管に直接NPを導入し、適用することによってNPの動きを狭い範囲で制御します同時に磁場。したがって、NPは血管内の血流に影響されることなく適切な位置に固定することができます。それ以外の場合、ドラッグデリバリーの対象となるNPには特定の制限しかありません。 NPを標的とすることは、化学療法薬の全身分布に影響を及ぼし、遊離腫瘍細胞および微小転移に対する化学療法の効果を減少させます。標的薬を装備している場合、標的効果が高まる傾向がありますが、既存の研究では改善は明らかではありません。さらに、抗腫瘍薬だけですべての腫瘍幹細胞を排除することはできません。それにもかかわらず、NPの物理的特性に基づく理学療法は腫瘍幹細胞に対してより効果的である傾向があります。したがって、将来的には、凍結手術、光熱療法(PTT)、光線力学療法(PDT)など、薬物キャリアを標的とする多機能NPを使用して、腫瘍治療用の多機能NPを形成することが推奨される傾向があります。
凍結手術用ナノ粒子
凍結によって腫瘍組織を破壊する技術である凍結手術は、侵襲性が低く、コストが低く、術中の出血が少なく、術後の合併症が少ないという利点がありますが、凍結効率が不十分で周囲の組織が凍結損傷するなどの欠点があります[28]。不凍タンパク質(AFP-1)などの保護剤がコールドアブレーションを支援するために利用されてきましたが、その効果はまだ理想的ではありません[72]。ナノテクノロジーの発展に伴い、ナノ凍結手術の概念が提案されました。ナノ凍結手術の基本的なメカニズムは、特定の物理的または化学的特性を持つNPを腫瘍組織に導入することです。 NPの特性を利用することで、凍結の効率と効果を向上させるだけでなく、氷球形成の範囲調整と方向を制御することもできます。したがって、ナノ凍結手術は、腫瘍組織を殺し、周囲の健康な組織が同時に凍結するのを防ぐことができます[73]。ナノ凍結手術の利点を図5に示します。
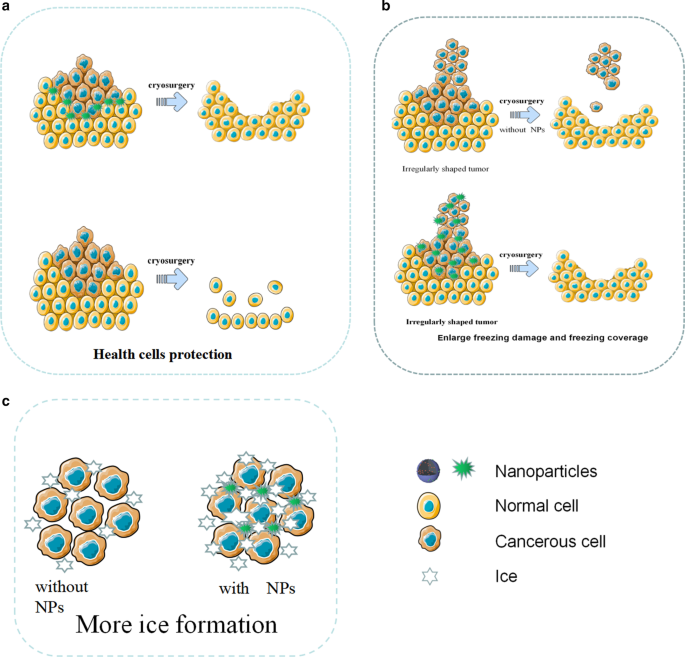
凍結手術用のNPの図解。 a NPは凍結手術中に健康細胞を保護します。 b NPは、凍結による損傷を強化し、凍結範囲を制御します。 c NPの助けを借りて、より多くの氷が形成されました
凍結手術では、細胞内の氷の形成が腫瘍細胞の損傷の鍵となります。一方、研究は、NPが細胞内の氷の形成を効果的に誘発できることを証明しています[28]。外部粒子としてのNPは、不均一な核形成を引き起こす可能性があります。研究によると、NPが豊富な組織は、従来の組織よりも急速に凍結し、不均一な核形成を起こしやすいことがわかっています。同じ凍結条件下で、NPによる組織の氷形成はより簡単であり、NPが細胞内の氷形成の速度と確率を大幅に高め、腫瘍細胞をより効果的に殺すことができることを示しています[74]。さらに、金属酸化物を含むNPは、腫瘍組織の熱伝導率を大幅に改善します。たとえば、LiuとDengは、NPがある場合とない場合の豚肉組織の温度応答曲線を比較しました。彼らは、NPを含む組織が急速に冷却され、最低温度が115℃に達する可能性があることを発見しました。これは、NPを含まない対照群よりもはるかに低い温度でした。
腫瘍は通常形状が不規則であるため、従来の凍結手術によって生成された氷の結晶は、すべての腫瘍組織を覆うとは限らない傾向があります。従来の凍結手術と比較して、ナノ凍結手術は問題に簡単に対処することができます。 NPは細胞外液に浸透し、熱伝導率などの優れた物性を持っているため、NPの分布によって氷球の成長方向や方向を制御することができます[73]。
凍結手術では、不十分な凍結は腫瘍組織を完全に破壊しない可能性があり、過度の凍結は隣接する健康な組織に損傷を与える可能性があります。特に腫瘍が壊れやすい臓器と密接に接触している場合、その位置が深い場合、またはその形状が不規則である場合、健康な組織への損傷は特に深刻になる可能性があります。近年、NPから作られた相変化材料(PCM)は、凍結手術中に周囲の健康な組織に対して優れた保護能力を示しています[75]。たとえば、Lv etal。潜熱が大きく、熱伝導率が低いマイクロカプセル化された相変化NPは、凍結手術の前に、腫瘍周辺の健康な組織にマイクロカプセル化された相変化NPを注入し、健康な組織への低温損傷を回避することを発見しました[76]。
NPは凍結手術で広く使用されていますが、それでも一連の欠陥があります。第一に、それはまだインビトロでNPを制御することができず、その結果、腫瘍組織におけるNPの不均一な分布および不十分な期待される機能をもたらす。第二に、さまざまな磁性ナノ粒子がありますが、invitro磁場制御NPの実際の効果はまだ理想的ではありません。さらに、ナノ凍結手術は臨床実験研究が不足しており、多くのNPはまだ実験段階にあります。
コールドアブレーションでのNPの適用は、一般に2つのタイプに分けることができます。相乗効果と保護効果です。これらはNPの設計要件とinvivoでの分布の点で異なります。将来的には、ナノ凍結手術はさまざまなNPによって支援される可能性があります。つまり、相乗的なNPは腫瘍内に分布し、保護的なNPは腫瘍の周囲に分布します。さらに、放射性粒子の移植前に腫瘍の位置決めに現在使用されている穿刺設計の3Dプリントコプレーナテンプレート(3DPCT)など、多くのナノ位置決めデバイスを凍結手術で使用できます。凍結手術の前に、保護NPに穴を開けて腫瘍の周囲に注入し、3D印刷コプラナーテンプレート(3DPCT)とCTガイダンスによって周囲の健康な組織を保護することができます。 NPは、凍結手術のアイスボールが腫瘍の不規則な端を覆うのを助けることができます。次に、相乗的なNPが、事前に設定されたアブレーション部位の穿刺または血管介入によって腫瘍組織に導入され、コールドアブレーションが実行されます。このナノ凍結手術技術は、不規則な腫瘍の低温焼灼の困難を克服するだけでなく、低温焼灼の効果を高め、健康な組織への損傷を減らすことができます。この方法は、ナノ凍結手術の将来の研究の方向性になる可能性があります。表4は、凍結手術で使用されるNPに関する最近の例を示しています。
<図> 図>PTTおよびPDT用のナノ粒子
現在、ナノ粒子(NP)に基づく光線熱療法(PTT)と光線力学療法(PDT)は、腫瘍治療中の強力な有効性、小さな浸潤、軽度の副作用という長所を示しています(図6)[80]。腫瘍細胞を直接殺すだけでなく、PDTおよびPTT治療によって生成された死んだ腫瘍細胞の断片を潜在的な抗原として使用して、光熱および光線力学免疫療法と呼ばれる継続的な免疫応答を引き起こすことができます[81]。 PTT処理の概念に基づいて設計されたナノ粒子は、新しいタイプの光から熱への変換ナノ材料であり、光エネルギーを熱エネルギーに変換して癌細胞を殺すことができます。従来の光熱変換材料と比較して、ナノ粒子には多くの利点があります。第一に、NPは粒子表面修飾を通じて腫瘍標的凝集の効果を達成することができ、これは標的腫瘍のより高い濃縮能力に貢献します[82、83]。第二に、ナノ粒子は、CT、MRI、および光音響イメージングによって正確に配置できる従来の光熱材料よりも優れたイメージング機能を備えています[84、85]。パンらによって合成された標的ナノ粒子。 0.2 W / cm 2 未満でPTTを実行できます NIRは、腫瘍細胞の核DNAを破壊し、DNA修復プロセスを阻害することによって腫瘍細胞のアポトーシスを誘導します[86]。表5に、PDTおよびPTTで使用されるNPに関する最近の例をいくつか示します。
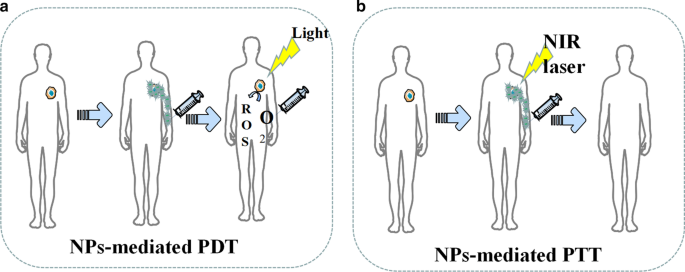
NPを介したPDTおよびPTTの図解。 a NPは活性酸素の生成を促進します。 b NPはPTT中の腫瘍損傷を増強します
さらに、いくつかの研究では、ナノ粒子を介したPTTが腫瘍の多剤耐性(MDR)を逆転させることができることがわかっています。薬物トランスポーター、多剤耐性関連タンパク質1(MRP1)、およびp-糖タンパク質(p-gp)の過剰発現は、さまざまな腫瘍でMDRを引き起こすと一般に考えられています[95]。たとえば、Liらによって設計された多機能の光トリガーナノ粒子。 PTTでのMRP1の発現を阻害する可能性があり、その結果、A549R細胞の薬剤耐性が逆転します[96]。王ら。金ナノ粒子と炭素ベースのナノ粒子の両方が、PTTでの熱ショック因子三量体の発現を促進し、それによってp-gpの生成を阻害することによってDOX耐性を克服できることを報告しました[97、98]。さらに、ナノ粒子を介したPTTは、腫瘍細胞膜の完全性を破壊することにより、化学療法の有効性を高めることもできます[99]。
PDT is a treatment that uses the selective retention of photosensitizing substances (PSs) in tumor tissue under the activation of specific wavelength excitation light and the presence of molecular oxygen to produce singlet oxygen and other reactive oxygen species, which leads to tumor cell apoptosis and necrosis [100]. However, traditional PS has poor tumor targeting, poor solubility, and instability, which is vulnerable to the internal environment [100]. Nanoparticle carriers modified by targeted molecules can not only improve the stability and biocompatibility of PS but also deliver PS to target cells, which improves the efficacy and reduces adverse effects [100]. Additionally, some common nanomaterials, like gold nanorods, have excellent PTT effects themselves. For example, Vankayala et al. found that the exposure of gold nanorods to near infra-red light (915 nm) were able to efficiently induce the generation of singlet oxygen [100].
In recent years, the role of up-conversion (UC) nanoparticles in PDT has attracted much attention. The NPs can convert long-wavelength light excitation into multiple short wavelengths, which enables the UC to replace the traditional ps-dependent short-wavelength excitation light with the near-infrared light with strong tissue penetration ability [101]. For example, Li et al. developed dual-band luminescent lanthanide nanoparticles as a PS carrier. This UC nanoparticles rely on the excitation light wavelength of 808 nm to achieve image-guided PDT without affecting imaging signals [102].
Since most photosensitive materials utilized in the phototherapy are metals, the biocompatibility of NPs designed for inorganic nanomaterials like metal ions still needs to be improved.
NPs-mediated phototherapy is now credited for not only the effectiveness against tumor but also the potential for spare internal space of nanoparticles since the therapy only utilizes the physical properties of NPs skeleton. Therefore, NPs are often multifunctioned by PDT and PTT. In the future, such NPs may be designed as dedicated NPs for tumor stem cells that are not sensitive to chemotherapy. Tumor stem cells are dormant for a long time and have a variety of drug-resistant molecules, so it is difficult to kill them by conventional treatments like chemotherapy, whereas the light therapy is more effective by killing the tumor stem cells physically. In the future, nanophysical therapy may be used with many other techniques, such as the multifunctional NPs for photothermal therapy after cryosurgery. Multifunctional NPs mediated therapy can give full play to its characteristics of low side effects, strong local lethality, and tumor stem cell killing. In addition, because nano-physiotherapy has a local killing effect and can effectively kill tumor stem cells, it may become a treatment method for small metastases.
Nanoparticles for Radiotherapy
Radiotherapy (RT) is a tumor treatment technique that kills local cells by ionizing radiation generated by rays and is currently an effective treatment for many primary and metastatic solid tumors [103]. Experiments prove that radiotherapy can effectively kill tumor stem cells [104].However, how to further improve the efficacy of radiotherapy is still a serious challenge. In recent years, nanoparticles in the field of radiotherapy have demonstrated strong radiosensitization capabilities, tumor-targeted delivery capabilities of radiosensitizing drugs, and imaging guidance enhancement capabilities [105]. At present, the most popular nanoparticles are made by high Z (atomic number) metal materials, which are featured by chemical inertness and strong radiation absorption capacity. They produce various reactions such as photoelectric effect and Compton effect after absorbing radiation, thereby releasing a variety of particles such as optoelectronics, Compton electrons, and Auger electrons. These electrons react with organic molecules or water in tumor cells to generate a large number of free radicals, leading to synergistic chemotherapy [106]. Common chemotherapy-sensitized NPs are currentlycategorized as precious metals, iron oxides, and semiconductors in terms of materials.
Precious metals NPs are made of high atomic number metal materials such as gold, silver, gadolinium, hafnium, platinum, bismuth, etc. [107]. Among them, gold nanoparticles have become the most popular NPs due to their good biocompatibility, chemical stability, and relatively strong photoelectric absorption coefficient [108]. In 2000, Herold et al. discovered the chemosensitizing ability of gold nanoparticles in kilovoltage X-rays. Nowadays, the specific mechanism of chemosensitization of gold nanoparticles is not yet clear, and the mainstream view believes that it depends on the photoelectric absorption capacity of high atomic number [109]. In addition to this, there are studies suggesting that the presence of gold nanoparticles improve the chemical sensitization of DNA to radiation, which increases the DNA damage induced by ionizing radiation (IR). At the same time, gold NPs can catalyze the mechanism of radiotherapy sensitization such as free radical production [105]. For instance, Liu found that AuNPs could significantly increase the production of hydroxyl radicals as well as the killing effect of x-rays and fast carbon ions on cells [110]. The hypothesis of the chemotherapy sensitization mechanism of other precious metals is similar to that of gold nanoparticles. Particularly, platinum NPs have an anti-tumor effect due to the inherent nature. Consequently, platinum NPs are expected to play the role of chemotherapy and radiotherapy simultaneously. However, the number of relevant research reports is insufficient, and the sensitizing effect of platinum NPs is also questionable. For example, Charest et al. reported that liposomal formulation of cisplatin was able to increase the uptake of platinum by tumor cells, and could enhance the killing of F98 glioma cells by γ-rays at the same time [111]. On the contrary, Jawaid et al. reported that platinum NPs would reduce the generation of reactive oxygen species (ROS) and the efficacy of radiotherapy during chemotherapy [112].
Iron oxide nanoparticles (IONs), especially the superparamagnetic magnet Fe3 O 4 , have shown great potential in image-guided tumor radiotherapy because they are capable of enhancing the dose of radiotherapy and MRI imaging, whereas its sensitization mechanism is not clear yet. Its sensitization mechanism is not yet clear. Some studies believe that iron oxide NPs mainly catalyze the generation of ROS through Fenton's reaction and Haber–Weiss reaction. Then the highly reactive ROS will kill tumors [112,113,115]. Other studies propose that the mechanism depends on the radiation sensitization and synergistic effects of magnetic nanoparticles. As Khoei reported, iron oxide NPs can improve the radiosensitization of prostate cancer cells in vitro [116]. Huang et al. pointed out that cross-linked dextran-coated IONs (CLIONs) could be internalized by HeLa cells and EMT-6 mouse breast cancer cells, which enhances radiation therapy [117]. Although the synergistic effect of iron oxide NPs is obvious, its biological safety still needs to be improved. Many studies have proved that the biocompatibility and chemical stability of iron oxide NPs are questionable, and it has certain toxicity [118].
Semiconductor NPs like silica NPs have also been found to have a synergistic effect on radiotherapy. For instance, Zhang et al. used flow cytometry analysis and MTT experiments to find that mesoporous silica NPs can effectively enhance the radiotherapy of glioblastoma [119]. He et al. reported the mechanism of radioactive enhancement of silica NPs. He found that under X-ray irradiation, silica nanoparticles could produce fine hydroxyl radicals, which can effectively kill tumor cells [120].
At present, although many experiments have confirmed that NPs were able to sensitize radiotherapy, the specific mechanism of sensitization is still unclear, which hinders the development of new sensitized NPs. There are some doctrines like sensitizing chemotherapy that promotes free radical production. Nevertheless, there is a lack of a quantitative relationship among the amount of free radical production, radiation intensity, and physical data of nanoparticles. In addition, most sensitized NPs are made of high atomic number metals. These metals have many disadvantages in human body such as difficulty in self-metabolism and biodegrading. Meanwhile, long-term accumulation of the metals will produce toxicity, which limits the safe use of radiosensitized NPs. Moreover, compared with the radiotherapy sensitization NPs, fewer studies focused on NPs which can prevent the adverse reactions of radiotherapy and protect healthy tissues. The research on radiotherapy protective NPs is short in quantities.
In the future, searching for NPs material that can be metabolized by the kidney, biometabolized, biocompatible, stable in physicochemical properties, and inherently less toxic, or looking for surface modification that can help the body metabolize NPs may become a research direction for sensitized NPs. Moreover, although there have been many NPs studies on multi-function, namely simultaneous sensitization of radiotherapy and chemotherapy, there are still many potentials in this field, which are worthy of focus in the future. The development of protective NPs that can protect normal tissues around radiotherapy and alleviate poor defense against radiotherapy may also become a research direction.
結論
The poor curative effect, inefficient targeting ability, various side effects, and potential biological risk are some of the unfavorable attributes of conventional cancer therapy and diagnosis. In recent years, advanced nanotechnology and molecular cell biology have promoted the applications of NPs in cancer field. Not only metal NPs, but also many lipid, nucleic acid and silicon NPs showed evident outperformance in cancer diagnosis and treatment.. Moreover, new generation of NPs is no longer limited to solo but multiple functions. For example, gold-coated poly(lactic-co-glycolic acid) (PLGA) NPs equipped with PD-1 blockers which were designed by Luo et al. can not only target drug delivery but also mediate PTT therapy [121]. (Pd @ Au) / Fe3O4 Spirulina NPs with doxorubicin created by Wang et al. demonstrated the functions of photothermal therapy, delivery of chemotherapy drugs, and magnetic field control in cell experiments [122]. Multifunctional nanoparticles will become the trend of future research.
At present, we find that most of the nanoparticles only stay in vivo and in vitro stage. According to this review, we think the following reasons hinder the clinical application of NPs.
- (i)
Lack of injection routes and methods
Most NPs are injected into body via puncture or intravenous injection. Therefore, the blood flow will take away NPs, making NPs difficult to stay in the target area for a long time, which leads to just few NPs that can be uptaked by tumor cells. Low-concentration drugs cannot produce the expected therapeutic effect, and low-concentration NPs also affect the physical killing effects of PDT, PTT, cryosurgery, and radiotherapy. In our opinion, magnetic NPs platform may be a solution. There have been many in vitro and in vivo experiments that have proved the feasibility of using the three-dimensional magnetic field to control the movement of NPs against blood flow [122,123,125]. However, how to solve the interference of the human body to the magnetic field, how to solve the impact of blood cells colliding with NPs, and how to control a large number of NPs in a group are still in discovery.
- (ii)
Difficulty in localization of NPs in vivo
Compared with the human body, the size of NPs is too tiny. Even if NPs are loaded with fluorescent proteins, it is still difficult for conventional imaging equipment (CT, X-ray, MRI) to locate the NPs in the human body in real time. To deal with this challenge, photoacoustic computed tomography (PACT) may be a solution. Photoacoustic computed tomography (PACT) has attained high spatiotemporal resolution (125-μm in-plane resolution and 50-μs frame −1 data acquisition), deep penetration (48-mm tissue penetration in vivo), and anatomical and molecular contrasts [126]. Because of excellent performance, PACT has great potential in NPs localization imaging in vivo. The PACT-guided microrobotic system designed by Wu et al. has achieved controlled propulsion and prolonged cargo retention in vivo of NPs with a diameter of 50 μm [127]. Although the current resolution and deep penetration of PACT are still insufficient, it is superior to conventional imaging equipment (CT, X-ray, MRI) in terms of NPs imaging positioning.
- (iii)
Difficulty of degrading in the human body
Although NPs are made of high biosafety materials, there is still a risk of damages to liver, kidney, and other organs if they stay in the body for a long time and cannot be degraded or excreted The use of materials that will be disintegrated after near-infrared light irradiation to fabricate NPs may be a solution to this problem. Recently, more and more NPs have been produced by these materials. Such NPs mediate PTT while loading drugs, meanwhile, the substances produced by the disintegration of NPs can be rapidly metabolized by the human body. In addition, the use of more biocompatible and degradable materials for nanoparticle preparation is also a solution. For example, the surface of chitosan is positively charged and can be broken down by the colonic flora, which facilitates interaction with specific tissues and can be metabolized by the body. The biocompatibility and degradability of chitosan has been proven to be non-toxic at appropriate drug concentrations [128].
- (iv)
Difficulty in avoiding mononuclear phagocytic system (MPS)
In biofluids, NPs will adsorb proteins to form a corona layer referred to as “protein corona” in a broader sense giving biological identity to NPs and alters their biological characters, which will attract MPS especially macrophages to uptake NPs [129]. In order to avoid being uptaken by MPS, various polymer coatings such as forpolyether, polybetaine (PB) and polyolhave were investigated to cover NPs. For example, polyglycerol-grafting NPs are able to evade macrophage uptake by reducing protein adsorption [130]. In addition, there are two types of tumor-associated macrophages (TAM), M1 and M2. M1 macrophages inhibit tumor growth while M2 macrophages promote tumor growth. Therefore, no longer avoiding macrophages, but designing NPs targeted by macrophages, by regulating the function of macrophages, and even using macrophages as new drug carriers to exert anti-tumor effects may become a novel solution. At present, common design strategies for such NPs include inhibiting macrophage recruitment, depleting TAM, reprogramming TAMs, and blocking CD47-SIRPα pathway [131]. Among them, following the design concept of reprogramming or blocking CD47-SIRPα pathway, NPs that repolarize M2 macrophages to M1 type have made a breakthrough in vivo experiments [132].
Considering the above difficulties and referencing to advanced researches, we come up with a new possible design of NPs. The NPs skeleton is made of pyrolytic material (spirulina, exosomes, et al.). Then, photothermal materials (Au, Pd, etc.) are deposited on the NPs skeleton through electroless plating. After that the superparamagnetic iron oxide will be loaded on the surface of NPs through the sol–gel method. Then, suitable polymers (polybetaine, polyglycerol, etc.) will coat the NPs. Finally, drug (like doxorubicin) will be loaded on the NPs. Afterwards, under the guidance of PACT, NPs will be injected into the upstream of tumor supplying blood vessel, and the tumor will be irradiated with NIR. At the same time, three-dimensional magnetic field control is given to maximize the accumulation of NPs at the tumor site. Through this design, a large number of NPs will accumulate at the tumor site to ensure the drug concentration and PTT effect. At the same time, most NPs will be decomposed at the tumor site, and only a small number of NPs will circulate in the body.
Nowadays, anti-tumor therapy with NPs as the main body is still in the exploratory stage, and related technologies and equipments need to be invented, so it is unlikely to be clinically used in the short term. However, NPs can change part of the function or structure of many actual technologies. The upgrade of actual technologies is expected to be applied in clinic quickly, which contributes to upgrading the diagnosis and treatment of tumors in consequence. For example, NPs can help to develop electrochemical devices based on the interaction between ions and conductive polymers, such as organic electrochemical transistors (OFETs), electrolyte gated field-effect transistors (FETs), fin field-effect transistor (FinFETs), tunneling field-effect transistors (TFETs), electrochemical lab-on-chips (LOCs) [133]. These electrochemical devices are widely used in various tumor testing and diagnostic equipment. The use of NPs can help improve the accuracy of the equipment and reduce the detecting time. Many studies indicate that medical equipment using electronic components upgraded by NPs have been applied clinically [133,134,136].
Based on the evidence cited above, future research of NPs may not only focus on NPs themselves but also consider a feasible administration and efficacy assessing platform. In addition, the platform needs to be able to monitor immunotoxicity, the long-term toxicity, and neurotoxicity of NPs. As nanotechnology develops, if these problems were solved, NPs would be an ideal approach to upgrade cancer therapy and diagnosis.
データと資料の可用性
All data generated or analysed during this study are included in this published article.
略語
- NPs:
-
ナノ粒子
- PDT:
-
Photodynamics therapy
- PTT:
-
Photothermal therapy
- SPR:
-
Plasmon resonance effect
- Au NRs:
-
Gold nanorods
- SI-ATRP:
-
Surface-initiated atom transfer radical polymerization
- NIPAAM:
-
N-isopropylacrylamide
- US:
-
Ultrasound
- MSNs:
-
Mesoporous silica nanoparticles
- USMO:
-
Ultrasmall manganese oxide
- GEM:
-
Gemcitabine
- OINPs:
-
Oxygen/indocyanine green-loaded lipid nanoparticles
- PA:
-
Photoacoustic
- MPI:
-
Magnetic particle imaging
- MRI:
-
Magnetic resonance imaging
- SPIO:
-
Superparamagnetic iron oxide
- USPIO:
-
Ultra-small SPIO
- OCT:
-
Optical coherence tomography
- MMOCT:
-
Magnetomotive optical coherence tomography
- mAb:
-
Monoclonal antibody
- DOX:
-
ドキソルビシン
- 5-FU:
-
5-Fluorouracil
- FA:
-
Folic acid
- PTX:
-
Paclitaxel
- ROS:
-
活性酸素種
- EPR:
-
Enhanced permeability and retention effect
- EGFR:
-
Epidermal growth factor receptor
- BHC:
-
Berberine hydrochloride
- AFP-1:
-
Antifreeze protein
- PCMs:
-
Phase change materials
- 3DPCT:
-
3D printed coplanar template
- RCDs:
-
Amino-rich red emissive carbon dots
- COF:
-
Covalent organic framework
- ICG:
-
Indocyanine green
- HSA:
-
Serum albumin
- MDR:
-
Multidrug resistance
- MRP1:
-
Multidrug resistance-associated protein 1
- p-gp:
-
P-glycoprotein
- PSs:
-
Photosensitizing substances
- UC:
-
Up-conversion
- RT:
-
Radiotherapy
- PLGA:
-
Poly(lactic-co-glycolic acid)
- PACT:
-
Photoacoustic computed tomography
- MPS:
-
Mononuclear phagocytic system
- PB:
-
Polybetaine
- TAM:
-
Tumor-associated macrophages
- OFETs:
-
Organic electrochemical transistors
- FETs:
-
Electrolyte gated field-effect transistors
- FinFETs:
-
Fin field-effect transistor
- TFETs:
-
Tunnelling field-effect transistors
- LOCs:
-
Electrochemical lab-on-chips
ナノマテリアル
- 貴金属の3Dプリント–新しいアプローチ?
- 新しいロボット:経済的で最高級
- ボッシュ:AIとIoTが新しい問題解決アプローチを要求する理由
- 癌治療のためのナノ粒子:現在の進歩と課題
- コバルトをドープしたFeMn2O4スピネルナノ粒子の調製と磁気特性
- ICAをロードしたmPEG-ICAナノ粒子の調製とLPS誘発性H9c2細胞損傷の治療におけるそれらの応用
- 非常に効果的な標的膵臓腫瘍治療のための、血液循環が延長され、生体適合性が改善されたレスベラトロール負荷アルブミンナノ粒子
- ナノ粒子の跳ね返りに及ぼす弾性剛性と表面接着の影響
- 銀ナノ構造の合成方法と応用における最近の進歩
- アルミニウムおよびアルミニウム合金の熱処理
- 銅および銅合金の熱処理